Authors: Fred Veer, Ton Romein and Pim Buskermolen
Source: International Journal of Structural Glass and Advanced Materials Research, 6(1), 15-22.
DOI: https://doi.org/10.3844/sgamrsp.2022.15.22
Abstract
Neglecting the accidental soccer or golf ball hitting the window of the neighbors in a movie, actual impact on glass is rare. In architecture, the only significant case in the literature is where a fully tempered laminated panel of the New York Apple cube was hit by a small stone launched by a snow blower. Nowadays a lot of glass is used in sound screens next to highways. These are however subjected to regular impact of small, fastmoving hard bodies. Mostly small stones which are removed from the asphalt road surface due to wear and are launched by car tires. This is a regular occurrence leading to safety questions and also to considerable cost as replacing the sound screen panels not only costs money but also requires closing down at least the outer lane of the highway, thus reducing the traffic flow. Thus a study was made of the impact resistance of annealed, heat-strengthened, and fully tempered laminated glass using test panels. This was followed by the testing of a full-size sound screen panel. The results show that the impact resistance of tempered glass is determined by the amount of compressive surface pre-stress. However, it is also noted that although fully tempered glass better resists impact, it has no residual strength after an impact with the critical energy.
Introduction
Impacts on architectural glass are infrequent and only the single case of a stone launched by a snow blower hitting a fully tempered laminated glass panel of the apple cube in New York, (Quirk, 2021), is in the open literature. In the field of car windows and glass sound screens impact problems are however a real concern. Figure 1 shows an actual example of a fully tempered laminated glass sound screen panel after both glass plies failed. In certain cases, such as shown in Fig. 2, where a glass sound screen leans over a by-pass, safety concerns can lead to immediate removal of damaged glass.
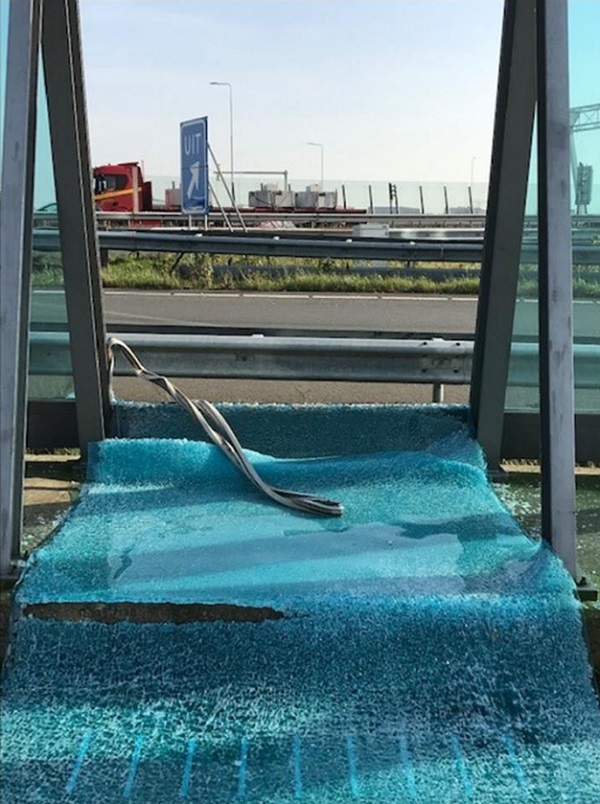
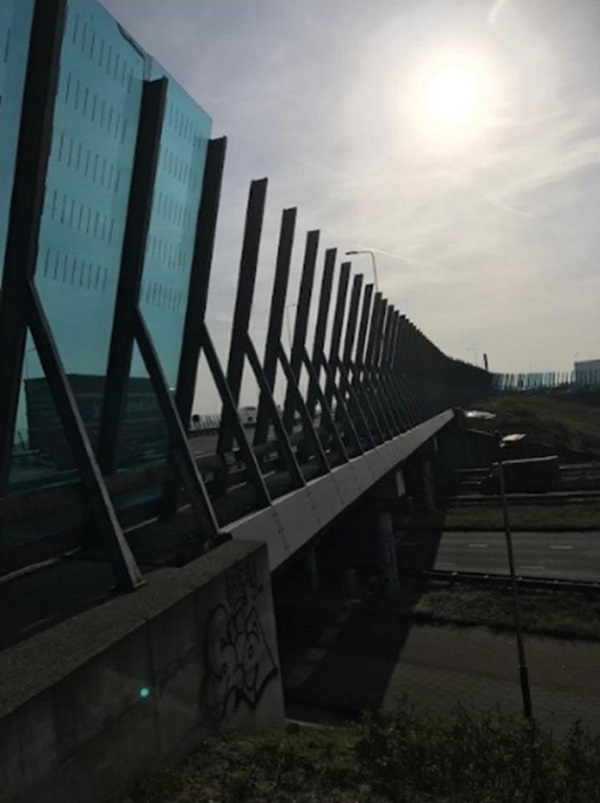
Although impact failures can be identified by the fracture pattern, (Bradt, 2011; Wagner, 2012), information about when glass cracks on small hard body impact is rare. Chu et al. (2018), have determined the velocity of small objects being launched from under car tires. This is essentially the speed of the car at moment of launch. Bradt (2011); Förch, (2020), have shown that the loading rate has an effect on glass strength, the high strain rates at impact should make the glass stronger. However small fast hard body impact on glass is much more complicated due to the induced Hertzian cone fractures, (Chaudhri 1989; 2015). Essentially glasses can crack if the impact causes a critical tensile stress inside the glass. Thus small hard body impact loading on glass differs from normal impact or slow bending loading.
Analytical models of small hard body impact have been made by (Horvát et al., 2012 and Chen et al., 2017). These help explain the specific fracture patterns caused by small hard body impact given by Bradt, (2011); Wagner, (2012). They do not however provide much useful information for the glass designer.
Considering the gap in the literature between theory and practical use an experimental investigation involving 1 × 1 m test panels of annealed PVB laminated glass, heat-strengthened PVB laminated glass, and fully tempered PVB laminated glass was planned. The glass in all cases was 8 mm thick and 2 PVB foils with a total thickness of 0.76 mm were used, in glass parlance 88.2 panels.
This was followed by a test on an actual full-size glass sound screen panel, dimensions 6 × 1 m, composed of two layers of 6 mm heat-strengthened glass with 0.78 mm PVB in between, in glass parlance a 66.2 panel.
Experimental Methods on Test Panels
Glass panels 1×1 m wide, composed of 2 layers of 8 mm glass and 0.78 mm PVB in the middle were produced. Annealed, heat-strengthened, and fully tempered glass were used. The panels were laid on standard steel frames used to support sound screens. Rubber profiles were initially used as an intermediary between the glass and the steel. The set-up is shown in Fig. 3.
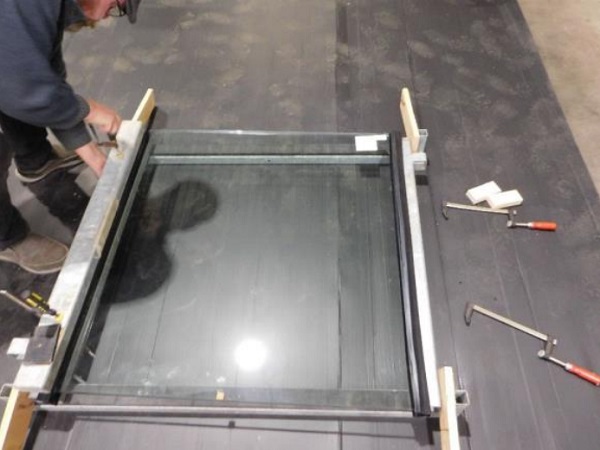
From a height of 5 m, steel spheres with diameters from 10 to 25 mm were dropped onto the glass panels. The spheres were aimed at the middle of the specimen and usually fell within a 300 mm circle around the geometrical center unless deliberately aimed at the edges. The dropping sequence started with the smallest size, bigger spheres being used for each successive impact. The speed at impact is 10 m/s or 36 km/h, which is the sideways speed component of a stone being launched by a car at an angle of 30° to the length axis of the sound screen. The calculated impact energies are given in Table 1.
It should be noted that the jump in kinetic energy relates to the 3rd power of the diameter of the spherical impactor. As these are only available in 1 mm increments, the possible increments in kinetic energy are non-linear.
Table 1: Kinetic energy of spherical steel impactors
Experimental Method for Actual Glass Sound Screen Panel
A single glass sound screen panel was provided by the Dutch road authorities. This was placed on foam blocks supported on wooden pellets. The same spherical steel impactors were dropped from a height of 5 m at different points of the panel. The test set-up is shown in Fig. 4.
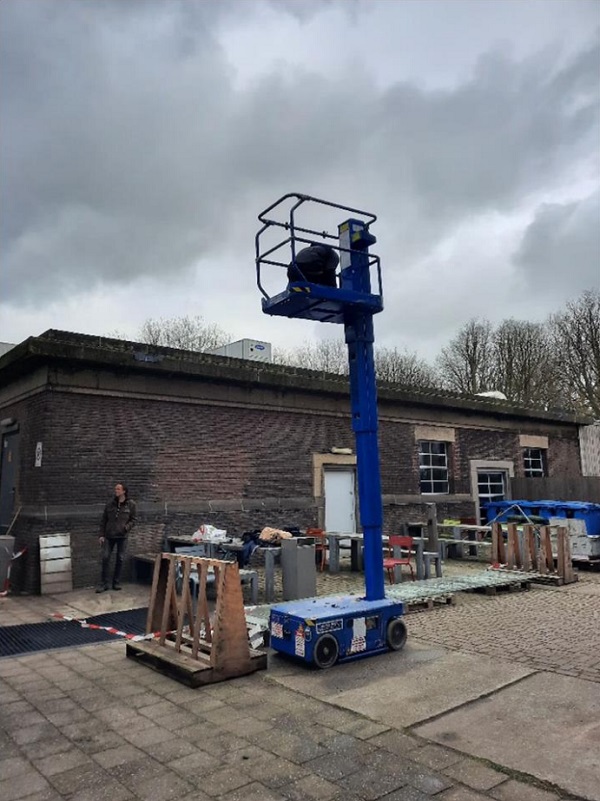
Measured Pre-Stresses
The compressive surface pre-stress in the glass panels was measured using a scalp 5 polarized light laser scanner. The results, which are the average of 5 measurements, are summarized in Table 2.
Table 2: Measured compressive surface pre-stress
Results of Impacts on Small Glass Panels
The results are given in Table 3. In annealed glass small hard body impact has no visible effect. Impacting spherical impactors of 14 or 15 mm diameter caused a cone crack, similar to those described by Chaudhri (1989; 2015). Larger impactors cause larger cone cracks, shown in Fig. 5. Cracking from the cone crack region is not caused by the actual impact. Cracks grow from the cone crack zone later due to stress fields from later impacts close to the original damage zone.
Table 3: Result of small hard body impacts on small panels
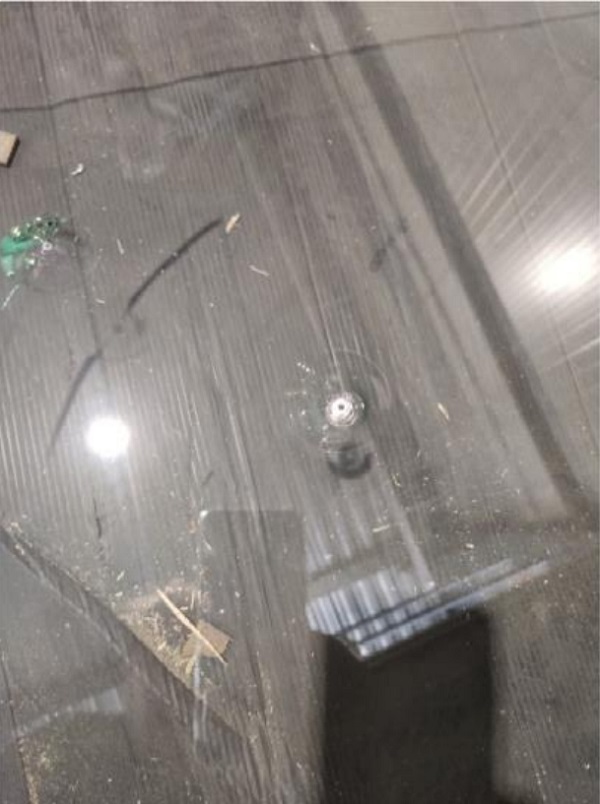
In heat-strengthened glass, the impact of a 16 to 18 mm diameter spherical impactor causes a cone crack with immediate cracking extending from it as shown in Fig. 6. Additional impacts by larger impactors cause more damage. In most specimens after 3 or 4 large cracks have been made, larger impactors do not cause extra damage, presumably as the decreased stiffness reduced the impact stress. One specimen was turned upside down after testing, with the back face being tested also. This specimen could, even after multiple impacts on both sides, still carry a large static load safely as is shown in Fig. 7. As shown by Veer et al. (2021), heat-strengthened glass retains at significant fraction of its residual surface compressive strength after fracture, so this is not surprising.
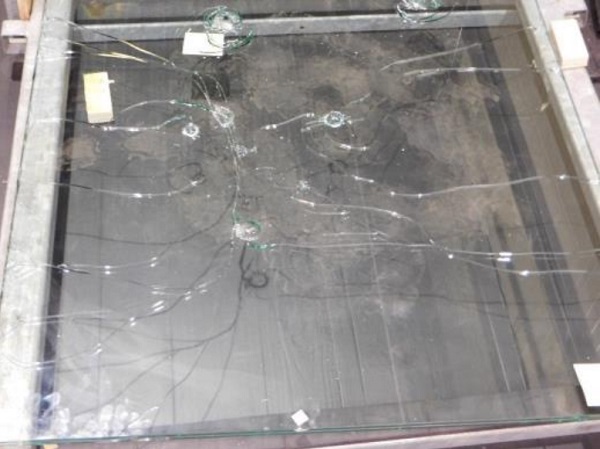
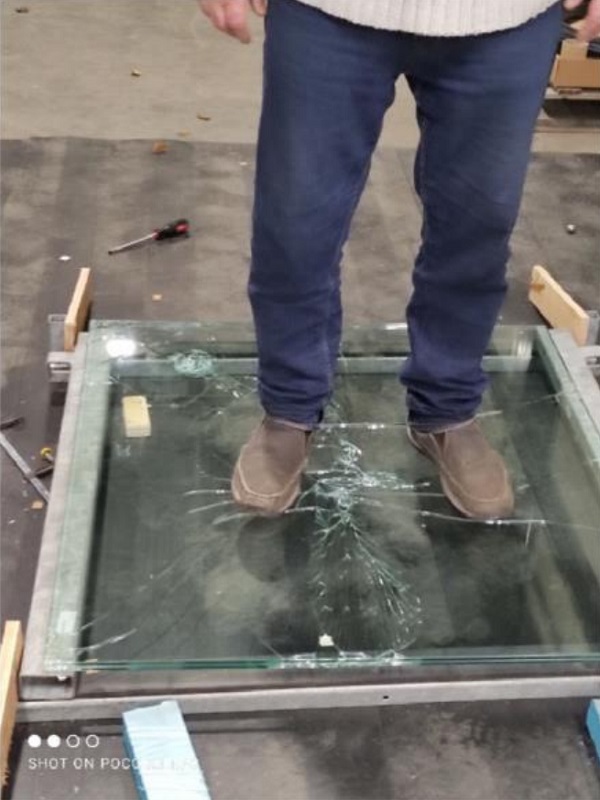
In fully tempered glass with sub-critical impactors slight chipping of the surface, as shown in Fig. 8 is observed. This is not noticeable in annealed and heat-strengthened glass. Larger impactors of 20 mm diameter or more cause total failure of the top layer. An example is shown in Fig. 9. Additional impact of a 25 mm diameter spherical impactors caused failure of the bottom plate also in some cases, leading to total structural failure of the fully tempered panel, as shown in Fig. 10.
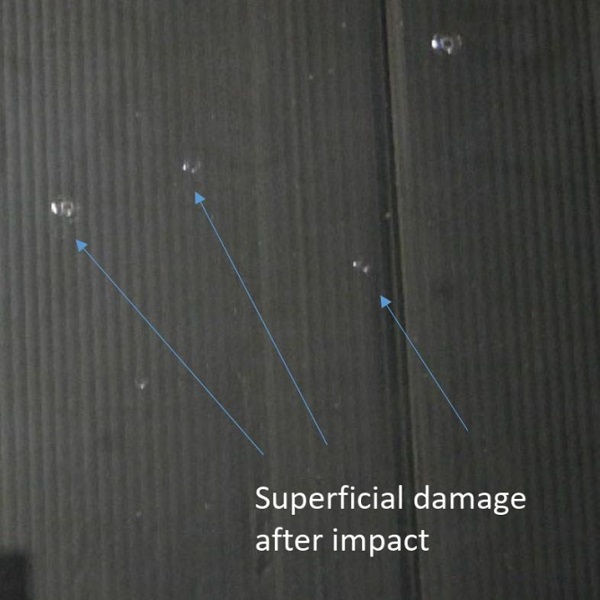
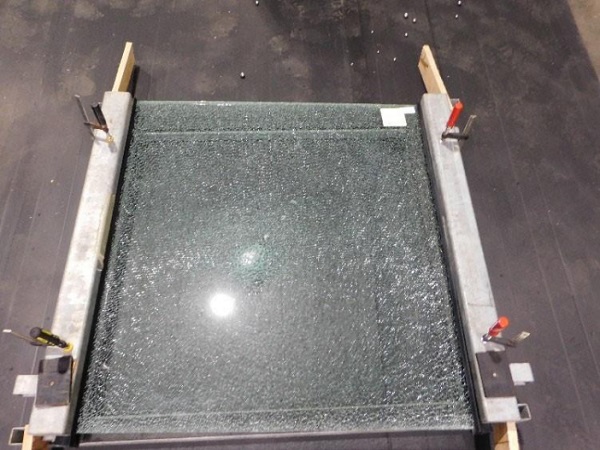
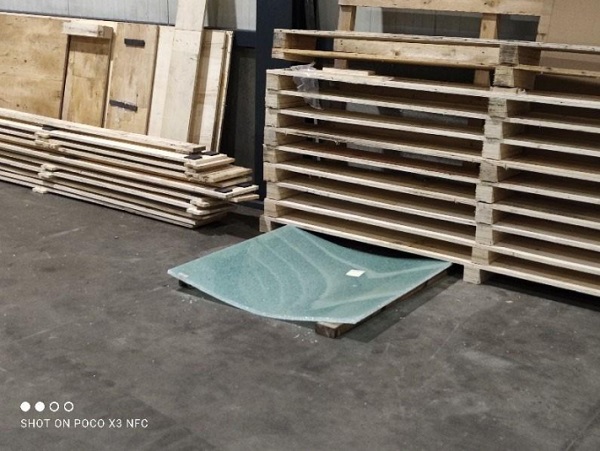
Results of Impacts on Full Scale Sound Screen Panel
Tests were done at 6 different locations of the panel, varying in support and aiming at the middle or edge of the panel. The results are described for each location in Table 4 and Fig. 11, 12 and 13 show the impact damage.
Table 4: Tests on full scale sound panel (66.2 HS)
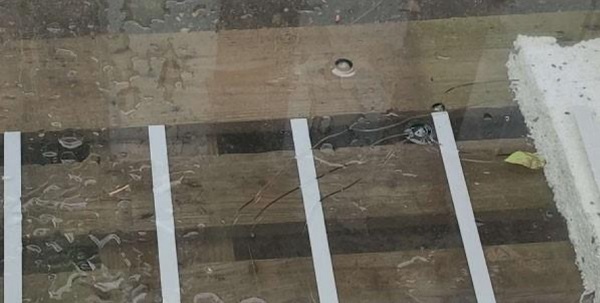
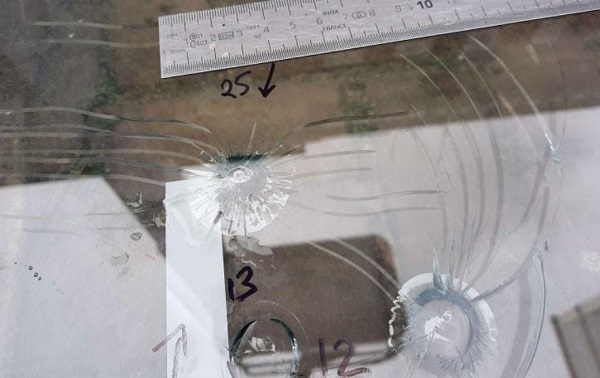
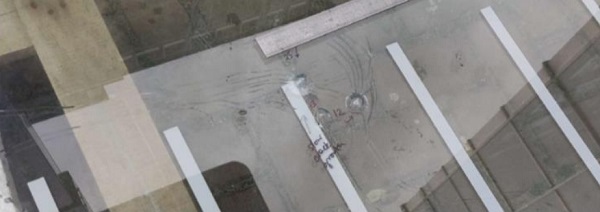
Discussion
Looking at the 1 × 1 m specimens the results show that fully tempered glass is the most resistant to impact damage. However, if the threshold for impact damage is exceeded the pane completely disintegrates. The tests however also show that a large impact on the fragmented pane can still cause the back pane to fragment also. In that case, as is clear from Fig. 9, there is no residual safety and the damaged panel will not be retained within its frame.
The annealed glass has good impact resistance in the sense that impacts only cause cone crack damage. However, any tensile stress from subsequent impact, or presumably wind or other out-of-plane loading, will cause cracks to grow from the cone crack. In that sense the annealed glass is the worst choice in that non-critical damage quickly becomes critical damage. So there is effectively no residual strength after impact.
The heat-strengthened glass is demonstrably the most safe. Impact with an energy above the threshold for impact damage causes a cone crack to form which immediately cracks further, presumably due to the tensile stress in the middle of the glass. However multiple impacts, even on both sides, do not impair the safety as the damaged panel can still sustain a significant load as Fig. 10 shows.
Figure 14 shows a plot of pre-stress against impact energy causing damage for all tests, with a lower and upped boundary. Although the scatter is considerable it is clear that higher surface compression pre-stress causes a significant increase in the critical kinetic energy to cause impact damage. This however is outweighed for practical purposes by the lack of residual strength caused by the total fragmentation of fully tempered glass.
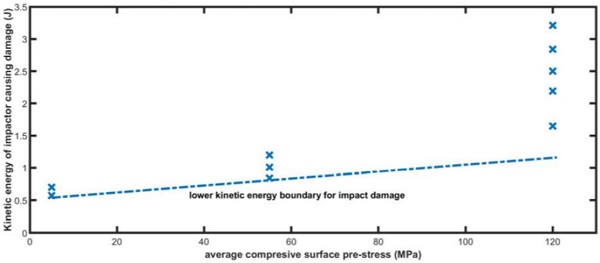
The results from the tests on the full-scale panel are more complex. The glass is thinner and the average surface compression stress is lower than in the 1 × 1 m panels. Where the glass impacted is locally supported by the foam supports the critical energy for impact damage exceeds that found for the 1 × 1 m panels. Where the glass is impacted between the foam supports the critical energy for impact damage is lower. As the 66.2 panel has about half the bending stiffness of the 88.2. Panels, apparently the increased flexure decreases the critical energy for impact damage. The reasons for this requires further research to elucidate. The most critical finding is however that the fullscale glass panel is very resilient to impact damage and has enough residual strength to maintain both structural safety and its sound screen function even after multiple impacts, even though the architectural function is partially lost.
Conclusion
From the results, the authors conclude that:
- The critical kinetic energy to cause impact damage on laminated glass panels increases with the surface compressive pre-stress in the glass
- Although fully tempered glass has the highest resistance to impact damage, it has effectively no residual strength after impact damage occurs
- Only heat-strengthened glass has sufficient residual strength after severe damage from multiple impacts to continue the safe functioning of a sound screen
- For glass sound screens thus only laminated heat-strengthened glass should be considered safe
- Increased support of the glass seems to increase the resistance to impact, although this needs to be examined further
Acknowledgment
The authors acknowledge the support of the Dutch road authorities “Rijkswaterstaat” and Sweco bv. In providing financing and test materials.
Author’s Contributions
Fred Veer: Supervised the whole process, wrote the reports and paper.
Ton Romein: Conducted the impact tests on the small panels.
Pim Buskermolen: Conducted the impact tests on the large panel.
Ethics
The research was funded by the Dutch road authority, Rijkswaterstaat, and Sweco B.V. The authors confirm that there is no conflict of interest.
References
- Bradt, R. C. (2011). The fractography and crack patterns of broken glass. Journal of failure analysis and prevention, 11(2), 79-96. https://link.springer.com/article/10.1007/s11668-011-9432-5
- Chaudhri, M. M. (2015). Dynamic fracture of inorganic glasses by hard spherical and conical projectiles. Philosophical Transactions of the Royal Society A: Mathematical, Physical and Engineering Sciences, 373(2038), 20140135. https://royalsocietypublishing.org/doi/full/10.1098/rsta.2014.0135
- Chaudhri, M. M., & Liangyi, C. (1989). The orientation of the Hertzian cone crack in the soda-lime glass is formed by oblique dynamic and quasi-static loading with a hardsphere. Journal of materials science, 24(10), 3441-3448. https://link.springer.com/article/10.1007/BF02385722
- Chen, S., Zang, M., Wang, D., Yoshimura, S., & Yamada, T. (2017). Numerical analysis of impact failure of automotive laminated glass: A review. Composites Part B: Engineering, 122, 47-60. doi.org/10.1016/j.compositesb.2017.04.007
- Chu, Y. J., Eun, H. J., & Lee, S. Y. (2018). Prediction of Damage to the Vehicle Underbody due to Stone Chipping. Applied Sciences, 8(10), 1805. https://www.mdpi.com/346872
- Förch, M. (2020). Time-Temperature Dependency of Laminated Glass Subjected to Blast Load-A Numerical Study. International Journal of Structural Glass and Advanced Materials Research, 4(1), 69-81. https://pdfs.semanticscholar.org/3498/6ec373b2495f3ab30634d729744cf87de881.pdf
- Horvát, E. Á., Járai-Szabó, F., Brechet, Y., & Néda, Z. (2012). Spring-block approach for crack patterns in glass. Open Physics, 10(4), 926-935. https://www.degruyter.com/document/doi/10.2478/s11534-012-0030-2/html
- Quirk, V. (2021). The snow blower shatters a panel of apple cubes. https://www.archdaily.com/469821/snowblowershatters-panel-of-apple-cube taken from the internet.
- Veer, F. A., de Krom, D., & Nijsse, R. (2021). Demonstration of the Structural Resiliency of Damaged Sentryglas Laminated Heat Strengthened Glass Fins in Full-Scale Testing. International Journal of Structural Glass and Advanced Materials Research, 5(1), 29-37. https://research.tudelft.nl/en/publications/demonstration-of-the-structural-resiliency-of-damagedsentryglas
- Wagner, G. E. (2012). Wallner zeigt den Weg.