Authors: Ľubomír Šooš, Miloš Matúš, Marcela Pokusová, Viliam Čačko and Jozef Bábics
Source: Recycling 2021, 6(2), 26; https://doi.org/10.3390/recycling6020026
Abstract
Laminated glass is ever more frequently used nowadays. This applies to the automobile industry and the construction industry. In cars, this refers mostly to the front and rear windows, whereas in construction, technical safety glass is used for railings and window glass. The task of this type of glass is to provide sufficient resistance against mechanical impact and unpleasant weather conditions. At the same time, if it is damaged, it has to break into the smallest possible pieces, or, wherever possible, the glass should remain intact on the interlayer film to prevent shards from injuring people and animals in the immediate vicinity. The paper deals with the recycling of laminated glass, especially with the effective separation of glass (in the form of cullet) from the polyvinyl butyral (PVB) interlayer film.
The experimental research is focused on the mechanical separation of glass from the interlayer film by vibration, and also on the chemical cleaning of PVB film in order to allow subsequent recycling of both materials. The results quantify the efficiency of mechanical separation in the form of weight loss of the sample of laminated glass and define the particle size distribution of glass cullet, which is an important parameter in the possibility of glass recycling. The research leads to a methodology proposal for the separation of glass and PVB film and the design of equipment for this method.
1. Introduction
The significance of recycling glass is very great from the ecological, energy, and technical standpoints, and accordingly, glass is an important secondary raw material in the form of waste. According to data from The International Organization of Motor Vehicle Manufacturers (OICA), global car production is about 90 million cars per year. Assuming that one vehicle windshield contains approximately 13 kg of glass and 1 kg of PVB film, the total amount of glass for windshield production is approximately 1170 mil. kg and 90 mil. kg of PVB film per year. The total worldwide amount of PVB film produced for the automotive and construction industries is estimated at around 170 mil. kg per year [1,2,3,4].
Production lines of world-renowned manufacturers such as Solutia, DuPont, Sekisui, and Kuraray produce thousands of tons of PVB film per year for automotive and construction use, which is further pressed into laminated glass. Worldwide, 65% of all PVB films are used in automotive applications [1,4]. By-products from processed PVB films (5%) and trimmed films (less than 10%) must also be included in the total produced quantities. This represents a total amount of 105 million kg of waste of PVB films per year. According to the estimates of the Automotive Industry Association of Slovak Republic [5], each year in Slovakia, there is at hand available about 13,200 t of flat glass waste from the building trade and from automobile glass, around 3600 t.
Researchers and recycling companies are increasingly focusing on the recycling and recovery of end-of-life vehicle (ELV) materials. This is due to various strict government directives and environmental regulations, such as the Resource Conservation and Recovery Act (RCRA) of the USA [6], K-REACH [7], and the EU directive on ELV [4]. European directive number 2000/53/CE represents a challenge for the automotive industry since it sets a recovery limit of 95% by weight of ELV, of which 85% by recycling. The automotive industry supports these efforts in all EU countries. However, material recycling has generally been oriented to materials such as steel and aluminum and not on less attractive wastes such as glazing [8]. Clearly, it is necessary to significantly increase the efficient recycling of windshields as well. The removal of glazing is nonetheless given an explicit mention in the minimum operations obligations when ELV comes to dismantling [9].
The recovery priority should be the reuse of secondary raw material from the waste glass as input material for manufacturing new glass. When the secondary raw material fulfills the required technical parameters, the quality of the product is equal to a product manufactured from the primary material. By recycling glass waste, we save mainly primary material resources, energy, and water and avoid the overloading of landfills. Energy demands on the production of container glass fluctuate around 4.5–5 GJ per ton of molten glass [10]. If the proportion of shards in a batch increases by 10%, the energy intensity of the glass production will decrease by 2.5% (starting value is a 35% batch of shards). For container glass, the emission factor varies at present between 350 and 400 kg CO2/t of molten glass. If the batch contains 35% of shards, the volume of CO2 emissions is reduced by about 18.5%, and with a 60% share of shards, the volume of CO2 emissions is reduced by up to 32% [10].
The technology for manufacturing laminated glass depends on the usage of a type of interlayer. In the process of technical layering, elastic material with good adhesiveness to glass is placed between the glass panes. The materials usually used include polyvinyl butyral (PVB), ethyl vinyl acetate (EVA), or the ionoplast SentryGlassPlus (SGP). PVB is the most frequently used material for the manufacture of interlayer film in laminated glass. Laminated glass with film is usually produced under high pressure and temperature in an autoclave. Two glass planes are mostly joined by a film with a strong adhesive bond. However, this adhesion is an obstacle in the recycling process because the separation of the laminated film from the glass cullet causes the biggest problems.
It is possible to obtain clean glass by separation, but the polymer is often contaminated with glass or other foreign substances to such an extent that it is unsuitable for further use or recycling and ends up mostly in landfills. This is because the large glass content precludes burning it [2]. For perfect separation and achieving clean phases of the laminate, according to the literature [1,4,11,12,13,14], a wet method of separating the layers of glass from the film seems to be the only usable one. This uses the reverse effect of the connecting technology—with a decreasing mixture of the film, its adhesion to glass increases. The basis of the decomposition technology is therefore the decrease of the PVB adhesiveness through an increasing content of water in the film. The problem with this method is the economic effectiveness of the entire technological process.
Based on scientific publications and research reports in this field [1,4,9,13,15,16,17,18,19], the technology of combined separation must always be used for the effective separation of PVB film from the glass. This includes mechanical treatment by breaking the glass or separating the glass (either with or without breaking the film), followed by chemical separation under the thermal influence, and finally mechanical, e.g., hydrodynamic cleaning, washing, and drying. All the mentioned technological influences affect the properties of the recycled PVB. Therefore, it is necessary to pay attention to research into changes in the PVB properties during processing and their impact on recycling.
1.1. Use and Properties of Recycled Materials
Processed clear glass, in the form of cullet, can be reused in the manufacture of glass products, and it is a replacement for conventional raw materials. The glass industry enjoys two advantages by recycling glass cullet—cullet is relatively cheaper than the primary material (silica) and less electricity is used to melt it in the furnace [4]. In addition to these economic benefits, cullet recycling saves many natural resources.
The method of recycling and the applicability of cullets depend on their purity and fraction size. Larger clean cullets are immediately suitable in the glass industry for the production of glass products. The small size of the glass fractions in the form of glass powder can be successfully used for the production of foam glass as thermal insulation for buildings and acoustic insulation. This application is analyzed in detail in works [20,21,22]. The properties of this material are also investigated in detail in works [23,24]. Waste glass of various fractions and purity is widely used in the construction industry. Much scientific research deals with new perspectives of recycled glass in concrete, mortars, and building materials [25,26,27,28,29,30,31,32,33,34,35,36].
Experimental research [12] investigating the impact of recycling on the properties of PVB film has shown that the reuse of recycled PVB film in laminated glass is conditioned by quantification of the plasticizer loss that occurred during the windshield recycling and the preparation process of PVB blends. The amount of plasticizer affects the ratio of the viscosity of the dispersed phase and the PVB matrix, which in turn controls the morphology of PVB blends and their properties.
The results of scientific research [4] show the characteristics of recycled PVB, in comparison with new PVB, depend on the effect of temperature in order to determine the suitability for reuse. Recycled PVB changes due to temperature were evaluated by means of thermal gravimetric analysis (TGA). Research has shown that the stable decomposition of new PVB begins at 200 °C, but a rapid decomposition follows from 300 °C In contrast, recycled PVB has two different temperature ranges with rapid decomposition, i.e., 180–350 °C and 350–550 °C. The first rapid decomposition is caused by the decomposition of plasticizers in recycled PVB in the temperature range 180–350 °C. Very similar experimental results are reported by the study [1]. The average weight loss of recycled PVB, caused only due to the decomposition of plasticizers, was 13.62% in the temperature range 250–350 °C. In this study, the content of plasticizers in PVB was found to be 20 and 25% by weight by mass spectroscopy.
The investigated tensile properties of samples of new and recycled PVB film reported in work [1] were measured at room temperature on standard tensile samples in the form of a “dumbbell.” They were cut directly from the films. Recycled and new PVB had very similar properties, but recycled PVB achieved higher tensile strength. Research has shown that the recycled material did not differ significantly from the original PVB polymers since they had a similar chemical composition and weight fraction of plasticizer. Original and recycled material can be mixed together, while there were no incompatibilities between different varieties. Problems may arise due to the loss of plasticizer from recycled material depending on the selected processing conditions. However, the addition of dibutyl sebacate can compensate for this deficiency. It has also been found in the laboratory that recycled types of PVB can be used to laminate glasses without loss of optical purity.
1.2. Separation Technologies
Literature and patent studies have shown [4,37,38] that there are, in general, four valid methods for the separation of laminated glass waste—mechanical, thermodynamic, chemical, and combined methods. The most frequently used is the mechanical disintegration way, based on the principle of multiple disintegrations of glued waste and the consequent separation of the particles of glass and film. On lines for the recycling of laminated glass, purely mechanical or combined separation is mostly applied, depending on the required final product and its quality. From the standpoint of working media present in the separation process, the methods and their appertaining technologies can be grouped into two categories—the so-called dry (mechanical or thermodynamic separating) and wet methods (e.g., chemical separation).
The principle of mechanical separation is the subject of patent EP0249094 [11]. The essence of the patent is multiple cycles of combined disintegration and separation of fragments of the laminated glass.
Another mechanical separation variant is the American Patent US8220728 [39], which is primarily focused on the effective separating of recycled PVB film. The essence of the invention is that in the first step, multi-laminated glass is crushed and splintered in a cylindrical chamber equipped with crushing hammers of a specific shape, while the splinters fall through a screen to the bottom of the chamber. The problem of mechanical separation is also addressed by patent SK286370, which makes use of the principle of double rotating rollers situated around a longitudinal axis with a treated surface. One of the pair of rollers is placed immovably in a bearing structure, and the second is placed so as to be displaceable and adjustable. The pairs of rotating rollers are arranged vertically and behind each other at an established distance in the bearing structure, with the distance between the rollers of the following pair being less than that between the rollers of the preceding pair.
One example of a combined wet technology is the continuous line from the company Xinology Co., Ltd., Hong Kong, for the recycling of laminated glass and the separation of PVB or EVA film from glass residues. Apart from the mechanical technology, the line also uses flotation technology, a washer, and a dryer [40].
The Danish company Shark Solution A/S also successfully undertakes mechanical recycling, and their patented technology produces ground glass with a particle size of 0–5 mm containing less than 1% contaminated materials and films, allowing it to be deployed in high-performance applications [41].
Our institute, in cooperation with the company MAVEBA s.r.o [42], has developed equipment that acts as a prototype for recycling automobile glass. In contrast to the patented solution EP0567876 [37], the processing of the waste laminated glass takes place in equipment based on the principle of two rotating shafts with chains, at the ends of which are steel impact hammers. The ground mixture is shifted to a rotating sorter where it is further sorted into individual batches of glass shards and film. The output of the mill for side and back windows is 200 kg/h, while its total annual capacity with a two-shift operation is 1600 t. Compared to the EP0567876 patent, Maveba technology has hammers mounted on chains rather than rigid bars.
1.3. Conclusion of Analysis
From the results of the analysis, there arises one significant conclusion—that mechanical disintegration technology itself, along with the subsequent separation of the individual components of laminated glass, is cheap, but at the same time, the present technology is highly noisy and dusty. In addition, it does not facilitate the efficient recovery of waste. Even though there is interest in the film and chips, glass factories and chemical plants do not want to buy secondary raw materials that do not fulfill the required criteria for cleanliness. The recoverability of clean glass fragments and film available with currently available technologies is only 50–60%. The residue of the unseparated waste finishes up on landfills.
In combination with other technologies, efficiency and the purity of the individual components will surely increase, but the price for this technology will also rise sharply and remain economically unviable for small processing activities.
Accordingly, demand has arisen to develop a process and equipment that will be technologically acceptable and economically viable for small- and medium-sized enterprises with an annual capacity of 1000–2000 t. The aim of our experimental activities was the research and verification of a variety of separation principles.
1.4. Scientific Hypothesis
The basic scientific hypothesis that we adopted in the research for solving the given task was that the proposed technology should meet the requirement of keeping a “compact film” after the technological process of decomposition. In our opinion, in this way, we will achieve the highest degree of purity in the individual batches. This implies successfully designing a technology such that in the decomposition process disintegration of the glass waste does not occur. This process could take place in such a way that the separation of the glass could be achieved by breaking the glass and then by subsequently peeling and scraping the shards from the film so that the film remains as integral as possible.
2. Materials and Methods
2.1. Materials
For all the experiments described in this study, laminated glass was used in the form of windshields from the same car manufacturer brand. The tested windshields consist of two glass layers of a thickness of 2.5 mm and the PVB interlayer of a thickness of 0.76 mm. All the tested windshield samples were made from two float glass layers modified to heat-tempered glass and with a PVB interlayer of Trosifol® Standard.
2.2. Laminated Glass Breakage Tests
Breakage tests of laminated windshields and testing of the suitability of the tool shape for breaking were realized. Experimental work for testing various punch profiles was carried out in this stage. The breakage tests of the windshield samples (Figure 1) were conducted to determine the force at which the glass deformed and at what degree of force breakage occurred. Another very important output is the knowledge of which tool geometry (sharp or rounded) allows the glass to crack into fragments of identical size.
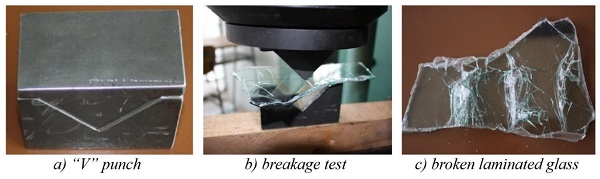
Two punches were used for the tests—the first was a “V” shape with a top angle of 90° (Figure 1a), and the second was an “S” cylinder shape (Figure 2a) with a radius of 50 mm. The sample size was ca. 100 × 70 mm. The usage of the V punch (Figure 1b) showed that the glass layer was to the greatest degree broken in the area of the punch edge, while the other parts of the glass sample were only slightly cracked (Figure 1c). It also remained firmly adhered to the film. A force of 0.05 kN was required to crack the glass, and a force higher than 2 kN was required to achieve full cracking in the pressing form.
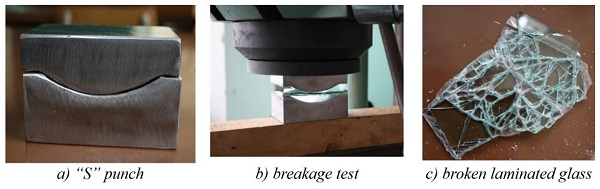
The “S” punch (Figure 2b) was simulated in conformity with the presumed cylinder profile of the future tool. Right from the first stroke of the press, the sample was more significantly cracked than with the V punch. This sample in the press was then progressively broken five times in succession, with the glass turned through 180° after each crunch (Figure 2c). The breaking force delivered onto the glass after each turning was always about 2 kN, with the exception of the final turning when the glass was so shattered that it bent under its own weight. The results of the testing on the “S” punch showed the glass stayed stuck to the film, even though it required very little force to release it. The output of these pretests is the value of the effective cracking force that is needed to crack the glass on pieces of similar shape and size without breaking the PVB interlayer. We, therefore, focused our further attention on finding accompanying operations or procedures to address the issue of separating the broken glass from the film.
The next step was an experiment for separating the broken glass by the action of heat. Pieces of dimensions of less than 5 × 5 mm could be easily mechanically separated from the film of PVB interlayer, but pieces of larger dimensions were harder to separate or could not be separated from the film at all.
The next step was to test the reaction of the broken windshield glass to heating with a hot air pistol and then rapid cooling in a liquid of temperatures down to −25 °C. The liquid was injected by syringe onto the broken glass, between the glass and the film layers. After heating the sample of crushed laminated glass and then injecting the frozen liquid (a solution of methanol and distilled water) on the border between the glass and the film, it became possible to separate the glass easily because the liquid reached under the individual fragments, as a result of which the final degradation of the adhesive strength occurred.
2.3. Vibration Tests
The second stage of the experimental work continued on the laboratory model of vibration equipment constructed for this purpose. The experimental equipment using mechanical vibration for grinding the samples of laminated glass is documented in Figure 3. The functional part of the machine is made up of two iron plates of 400 × 400 mm. The plates are connected together by a hinge at the bottom, and flexibly by springs on top. One plate is fixed to the base, while a vibrator (0.18 kW, 2880 rpm, centrifugal force 2.26 kN) is placed on the second plate. The working surfaces of both plates are made as a profile from rasterized horizontally placed cylindrical rods of 10 mm diameter.
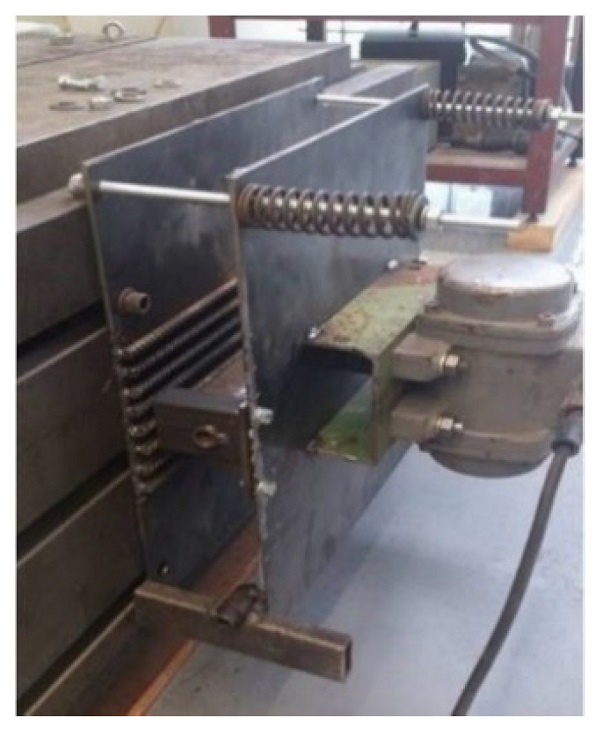
The actual measuring took place on three intact test samples of the windshield (Figure 4), each of 395 × 295 mm. Each sample was crushed by its own weight six times (three times from each side) while moving through the equipment. The samples’ weight was measured before the tests and after each individual crushing cycle. The measured values of the samples’ weight are shown in Table 1.
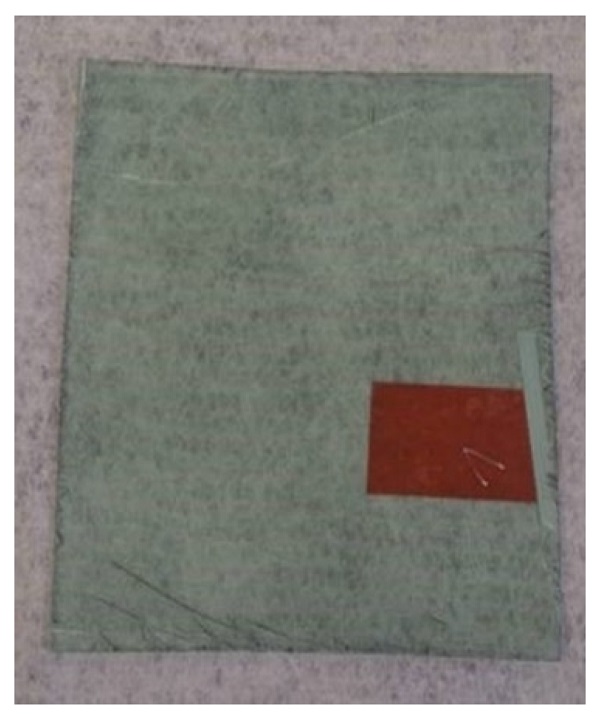
Table 1. Evolution of weight loss of analyzed samples over six vibration cycles.
The glass that peeled off the PVB film during the vibration cycles was captured in a collection vessel situated below the laboratory vibration equipment. The separated glass was subjected to granulometric analysis to determine the weight and proportion of glass in five-size cullet samples. To classify the glass particles, a Retsch AS 200 sieving device was used. The results of the granulometric analysis are presented in Table 2. From the table, it can be inferred that the highest weight ratio of cullet sample II with particles of size 2–4 mm is 36% in all the cases. The individual samples of glass after sieve analysis and the final PVB interlayer film are shown in Figure 5.
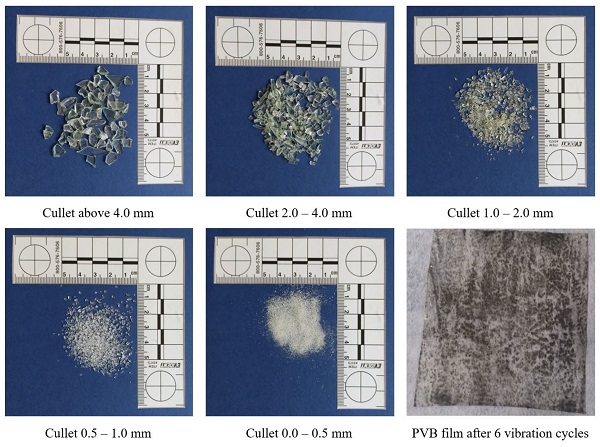
Table 2. Particle size analysis of cullet after vibration tests of glass samples 1 to 6.
3. Results and Discussion
3.1. Vibration Test
A summary and evaluation of the results attained from experimental measurements, the average of the original weight, the averages of the weights of the samples, and the development of average weight loss after each vibration cycle are shown in Table 1.
From Table 1, it follows that the average original weight of the selected sample of automobile glass, 1288 g, has been reduced by the mechanical separation method to a weight of 101 g, which represents a loss of weight of 92.16% and an approximately corresponding weight of PVB film (Figure 6). From the calculated average losses of weight, it is clear that the greatest loss took place after the first vibrations, when it was possible to watch larger cullet of glass falling from the PVB film, and the weight fell by about 408 g, i.e., 31.68%, from the original weight. On the other end of the scale, the least weight was lost after the fourth vibrating cycle, when the original weight fell by on average 98 g.
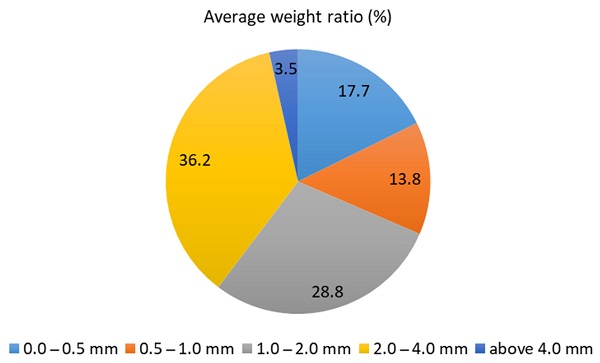
On the basis of known data on the PVB interlayer, such as its density ρ, film thickness glued into the automobile glass h, and the dimensions, it was possible to calculate the weight m of the original clean PVB interlayer as follows:
where
- ρ is the density of the PVB film; ρ = 1.07–1.2 g.cm⁻³; for the calculation, this was the lowest mean value;
- h is the thickness of the interlayer film in the automobile glass; h = 0.76 mm;
- a, b is the average dimension of the sample with PVB film; a = 395 mm, b = 295 mm.
The difference between the calculated film weight and the cleaned film weight is 6.25 g.
The measured and calculated values from the particle size analysis for all six samples subjected to mechanical recycling are shown in Table 2. The results of the analysis showed that the highest average value of separated glass weight, about 36.2%, was in size section II-cullet sample II, with parts from 2 mm to 4 mm, and the least, about 3.5%, occurred in size section I, with glass particles above 4 mm. The percentage share of the individual sections is illustrated in Figure 6.
3.2. Washing of Interlayer Film
The next test was focused on examining the possibilities for removing remaining glass fragments from PVB film following vibration (Figure 5) by the action of heat and a water solution CaCl₂. The prepared solution of water and CaCl₂ (700 mL H₂O, 280 g CaCl₂) was heated in a container to 60 °C. The PVB film was then soaked in the solution for a period of 5 min and washed by hand. The glass powder fragments were removed from the film by the hot solution and slid off. The quality of the surface cleanliness and the continuity of the recycled PVB film is documented in Figure 7.
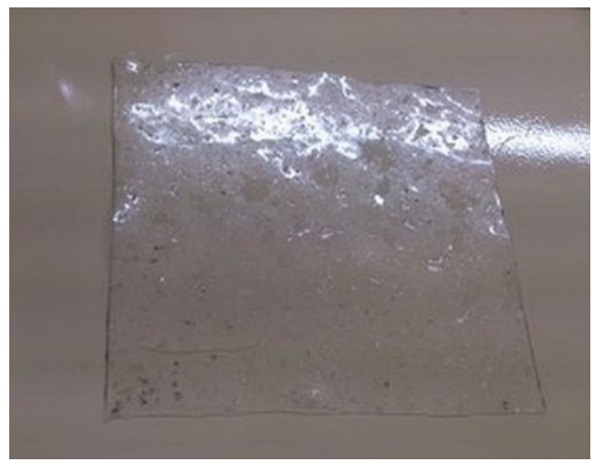
The weighing of the interlayer film after washing showed an average weight value of 96.72 g. The difference between the original weight of the primary film (94.75 g) and the film after washing is therefore +1.97 g. In an analysis of the recorded values, we can conclude that 99.85% efficiency in the decomposition of laminated glass by mechanical treatment and washing can be attained by the proposed technology.
4. Design of Technology Line Structure
The essence of construction in the design of the technological equipment for processing waste laminated glass resides in the fact that it is made up of a multistage set of variable modules. Due to this particular feature, it is possible on one device to process glued glass of various sizes and thicknesses, including multilaminated glass. In this way, new variable configurations for the specific requirements of different customers become possible. The described modules are capable of working independently or together with accompanying technology. Mutual movements and interaction can be linked up due to the frequency-controlled drive of individual rollers and the frequency of the selected industrial vibrator. On the one hand, the individual customer can acquire “tailor-made” machines, while, at the same time, their “modular conception” allows for the broad employment of such machines in the processing of waste laminated glass, mainly in the construction and automobile industries.
In the minimal configuration (Figure 8) [43], the line is made up of one breaking module (Figure 9b), in which the glass is broken in both transverse and longitudinal directions between two pairs of breaking profile rollers. The second line module is a vibration module (Figure 9c), in which a broken but compact car windshield can be shaken between a vibrating tool with pyramidal needles. The final module in this minimal makeup is the stripper module (Figure 9d) in which, on the basis of the various frequencies of the stripper rollers, mechanical cleaning of the PVB film is carried out.
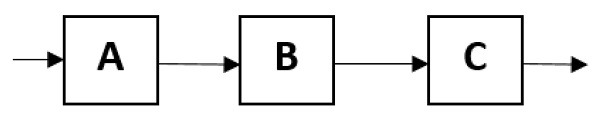
![Figure 9. Prototypes of decomposition line modules (utility design in SR No.SK8786). Reprinted with permission from ref. [44]. 2020, Owner: Slovak University of Technology in Bratislava.](/sites/default/files/inline-images/Fig9_143.jpg)
For thick and multi-laminated glass, any of the modules may be run a number of times (Figure 10). In the interest of increasing the efficiency of processing and the cleanliness of the final film product, the line configuration is finished up with reception tables by reception modules (Figure 9a) and washing modules for the cleaning of the PVB film in a heated water solution.

5. Conclusions
The main aim of the contribution presented here is a description of the research, development, and design leading to the construction of an effective high-efficiency technology for decomposition waste from multilayer glued glasses. On the principle of the proposed technology, it is possible to modify the optimal line configuration for economically efficient processing of laminated glass with an annual capacity of 500–2000 tons.
The proposed technology begins with the hypothesis that waste glued glass is not crushed in the process of processing but rather subjected to a decomposition process based on the principle of retaining the integrity of the PVB film. In comparison with classical mechanical technologies, the proposed technology is not as dusty or noisy and does not require such machine input. The principle of film “integrity” ensures the attainment of cleanliness with an adhering glass content of up to 100 ppm. This residual amount of glass is acceptable for the industrial recycling of PVB foils. PVB film separated in this way will be prepared for repeat extrusion and the production of new PVB film for laminated glass. Another significant advantage of the proposed technology is that it ensures the greatest cleanliness of the individual types of waste. An essential advantage of the proposed technology is the up to 99.85% yield of glass shards.
The multistage module technology for the processing of laminated glass may be modified simply according to the performance, type, and amount of waste to be processed. Finally, the design of the link also allows for a mobile arrangement.
6. Patents
The work reported in this manuscript results in one utility design in SR (SK8786) [44] and one patent application (SK: PP 107-2019): Method of Effective Recovery of Waste Laminated Glass and Modular Construction of the Device [43].
Author Contributions
Conceptualization, Ľ.Š. and M.M.; methodology, Ľ.Š.; validation, Ľ.Š. and M.M.; formal analysis, M.M. and M.P.; investigation, J.B. and V.Č.; resources, M.M.; data curation, Ľ.Š.; writing—original draft preparation, M.M. and Ľ.Š.; writing—review and editing, M.M.; supervision, Ľ.Š.; project administration, Ľ.Š.; funding acquisition, Ľ.Š. All authors have read and agreed to the published version of the manuscript.
Funding
This research was funded by the Slovak Research and Development Agency, grant number APVV-18-0505, and by the Ministry of Education, Science, Research, and Sport of the Slovak Republic under the Contract-University and industrial research and education platform of a recycling company (UNIVNET).
Data Availability Statement
The data presented in this study are available on request from the corresponding author.
Conflicts of Interest
The funders had no role in the design of the study; in the collection, analyses, or interpretation of data, in the writing of the manuscript, or in the decision to publish the results.
References
- Dhaliwal, A.K.; Hay, J.N. The characterization of polyvinyl butyral by thermal analysis. Thermochim. Acta 2002, 391, 245–255. [Google Scholar] [CrossRef]
- OICA. The International Organization of Motor Vehicle Manufacturers. 2014. Available online: http://www.oica.net/category/production-statistics/2014-statistics/ (accessed on 10 November 2018).
- Gorokhovski, A.V.; Escalante-Garcia, J.I.; Gashnikova, G.Y.; Nikulina, L.P.; Artemenko, S.E. Composite materials based on wastes of flat glass processing. Waste Manag. 2005, 25, 733–736. [Google Scholar] [CrossRef] [PubMed]
- Swain, B.; Park, J.R.; Shin, D.Y.; Park, K.-S.; Hong, M.H.; Lee, C.G. Recycling of waste automotive laminated glass and valorization of polyvinyl butyral through mechanochemical separation. Environ. Res. 2015, 142, 615–623. [Google Scholar] [CrossRef] [PubMed]
- Novotný, M. Recovery of Automotive Laminated Glass–I. Phase. Research Report; Slovak University of Technology in Bratislava: Bratislava, Slovak, 2015. [Google Scholar]
- United States Environmental Protection Agency (EPA). Wastes-Laws and Regulations; EPA: Washington, DC, USA, 1976.
- Ministry of Environment of Korea (MoE). Act on Registration and Evaluation of Chemical Substances “K-REACH”; Government Official Gazette no. 18021; 2013; pp. 48–68.
- Gerrard, J.; Kandlikar, M. Is European end-of-life vehicle legislation living up to expectations? Assessing the impact of the ELV Directive on ‘green’ innovation and vehicle recovery. J. Clean. Prod. 2007, 15, 17–27. [Google Scholar] [CrossRef]
- Farel, R.; Yannou, B.; Ghaffari, A.; Leroy, Y. A cost and benefit analysis of future end-of-life vehicle glazing recycling in France: A systematic approach. Resour. Conserv. Recycl. 2013, 74, 54–65. [Google Scholar] [CrossRef][Green Version]
- Slovak Glass Association (SGA). Search and Possibilities of Application of New Technologies in the Process of Waste Glass Treatment Which Cannot be Processed by Common Technologies-strategy of Research and Development of Technologies, Logistics and Processing of Individual Waste Streams-Research Report; Slovak Glass Association (SGA): Bratislava, Slovakia, 2015. [Google Scholar]
- Method and Plant for the Treatment of Laminated Glass. U.S. Patent EP0249094, 16 December 1987.
- Valera, T.S.; Demarquette, N.R. Polymer toughening using residue of recycled windshields: PVB film as impact modifier. Eur. Polym. J. 2008, 44, 755–768. [Google Scholar] [CrossRef]
- Tupý, M.; Měřínská, D.; Svoboda, P.; Zvoníček, J. Influence of water and magnesium ion on the optical properties in various plasticized PVB sheets. J. Appl. Polym. Sci. 2010, 118, 2100–2108. [Google Scholar] [CrossRef]
- Farel, R.; Yannou, B.; Bertoluci, G. Finding best practices for automotive glazing recycling: A network optimization model. J. Clean. Prod. 2013, 52, 446–461. [Google Scholar] [CrossRef][Green Version]
- Cheng, Y.W.; Cheng, J.H.; Wu, C.L.; Lin, C.H. Operational characteristics and performance evaluation of the ELV recycling industry in Taiwan. Resour. Conserv. Recycl. 2012, 65, 29–35. [Google Scholar] [CrossRef]
- Cumbul Altay, M.; Sivri, N.; Onat, B.; Şahin, Ü.; Zorağa, M.; Fatih Altay, H. Recycle of metals for end-of-life vehicles (ELVs) and relation to Kyoto protocol. Renew. Sustain. Energy Rev. 2011, 15, 2447–2451. [Google Scholar] [CrossRef]
- Suzuki, H.; Sato, K.; Ito, Y.; Kasai, M.; Morimoto, Y.; Nagao, T.; Ogawa, K. Research on dismantling technologies for improving ELV recycling. JSAE Rev. 2001, 22, 201–203. [Google Scholar] [CrossRef]
- Vermeulen, I.; Van Caneghem, J.; Block, C.; Baeyens, J.; Vandecasteele, C. Automotive shredder residue (ASR): Reviewing its pro-duction from end-of-life vehicles (ELVs) and its recycling, energy or chemicals' valorisation. J. Hazard. Mater. 2011, 190, 8–27. [Google Scholar] [CrossRef] [PubMed]
- Zhao, Q.; Chen, M. A comparison of ELV recycling system in China and Japan and China’s strategies. Resour. Conserv. Recycl. 2011, 57, 15–21. [Google Scholar] [CrossRef]
- Cozzarini, L.; Marsich, L.; Ferluga, A.; Schmid, C. Life Cycle Analysis of a novel thermal insulator obtained from recycled glass waste. Dev. Built Environ. 2020, 3, 100014. [Google Scholar] [CrossRef]
- Kyawoo D’Amore, G.; Caniato, M.; Travan, A.; Turco, G.; Marsich, L.; Ferluga, A.; Schmid, C. Innovative thermal and acoustic insulation foam from recycled waste glass powder. J. Clean. Prod. 2017, 165, 1306–1315. [Google Scholar] [CrossRef]
- Kyawoo D’amore, G.; Caniato, M.; Schmid, C.; Marsich, L.; Ferluga, A.; Cozzarini, L.; Marinò, A. An Innovative Thermal and Acoustic Insulation Foam for Naval Fire Doors Characterization and Study with FEM Analysis, Ebook-Technology and Science for the Ships of the Future; IOS Press: Amsterdam, The Netherlands, 2018; pp. 332–339. [Google Scholar] [CrossRef]
- Kourti, I.; Cheeseman, C.R. Properties and microstructure of lightweight aggregate produced from lignite coal fly ash and recycled glass. Resour. Conserv. Recycl. 2010, 54, 769–775. [Google Scholar] [CrossRef][Green Version]
- Ducman, V.; Mladenovic, A.; Suput, J.S. Lightweight aggregate based on waste glass and its alkali-silica reactivity. Cem. Concr. Res. 2002, 32, 223–226. [Google Scholar] [CrossRef]
- Calmon, J.L.; Sauera, A.S.; Vieira, G.L.; Teixeira, J.E.S.L. Effects of windshield waste glass on the properties of structural repair mortars. Cem. Concr. Compos. 2014, 53, 88–96. [Google Scholar] [CrossRef]
- Guo, P.; Meng, W.; Nassif, H.; Gou, H.; Bao, Y. New perspectives on recycling waste glass in manufacturing concrete for sustainable civil infrastructure. Constr. Build. Mater. 2020, 257, 119579. [Google Scholar] [CrossRef]
- Arabi, N.; Meftah, H.; Amara, H.; Kebaïli, O.; Berredjem, L. Valorization of recycled materials in development of self-compacting concrete: Mixing recycled concrete aggregates–Windshield waste glass aggregates. Constr. Build. Mater. 2019, 209, 364–376. [Google Scholar] [CrossRef]
- Lam, C.S.; Poon, C.S.; Chan, D. Enhancing the performance of pre-cast concrete blocks by incorporating waste glass–ASR consideration. Cem. Concr. Compos. 2007, 29, 616–625. [Google Scholar] [CrossRef]
- Sharifi, Y.; Houshiar, M.; Aghebati, B. Recycled glass replacement as fine aggregate in self-compacting concrete. Front. Struct. Civ. Eng. 2013, 7, 419–428. [Google Scholar] [CrossRef]
- Penacho, P.; de Brito, J.; Veiga, M.R. Physico-mechanical and performance characterization of mortars incorporating fine glass waste aggregate. Cem. Concr. Compos. 2014, 50, 47–59. [Google Scholar] [CrossRef]
- Tamanna, N.; Tuladhar, R.; Sivakugan, N. Performance of recycled waste glass sand as partial replacement of sand in concrete. Constr. Build. Mater. 2020, 239, 117804. [Google Scholar] [CrossRef]
- Chen, B.; Zhu, H.; Li, B.; Sham, M.; Li, Z. Study on the fire resistance performance of cementitious composites containing recycled glass cullets (RGCs). Constr. Build. Mater. 2020, 242, 117992. [Google Scholar] [CrossRef]
- Bostanci, S.C. Use of waste marble dust and recycled glass for sustainable concrete production. J. Clean. Prod. 2020, 251, 119785. [Google Scholar] [CrossRef]
- Khan, Q.S.; Sheikh, M.N.; McCarthy, T.J.; Robati, M.; Allen, M. Experimental investigation on foam concrete without and with recycled glass powder: A sustainable solution for future construction. Constr. Build. Mater. 2019, 201, 369–379. [Google Scholar] [CrossRef]
- Lu, J.-X.; Shen, P.; Zheng, H.; Zhan, B.; Ali, H.A.; He, P.; Poon, C.S. Synergetic recycling of waste glass and recycled aggregates in cement mortars: Physical, durability and microstructure performance. Cem. Concr. Compos. 2020, 113, 103632, in press. [Google Scholar] [CrossRef]
- Zeng, J.; Zhang, X.; Chen, G.; Wang, X.; Jiang, T. FRP-confined recycled glass aggregate concrete: Concept and axial compressive behavior. J. Build. Eng. 2020, 30, 101288. [Google Scholar] [CrossRef]
- Method and Apparatus for the Treatment and Recycling of Laminated Glass. Patent EP0567876, 3 November 1993.
- Priore, R. A patent intelligence analysis aimed at identifying eco-friendly methodologies for recycling PVB to be used as windscreens interlayer. World Pat. Inf. 2019, 59, 101932. [Google Scholar] [CrossRef]
- Method and Apparatus for Separating Glass and Plastic Foils in Laminated Glass. U.S. Patent 8,220,728, 17 July 2012.
- Available online: http://www.xinology.com/Glass-Processing-Equipments-Supplies-Consumables/glass-recycling/laminated-glass-recycling-system/introduction.html. (accessed on 15 October 2020).
- Available online: https://www.shark-solutions.com/glass-separation-tech (accessed on 15 October 2020).
- Šooš, Ľ.; Pokusová, M.; Matúš, M.; Rafaj, M.; Biath, P.; Ondruška, J.; Bábics, J. Mechanical and Chemical-Thermal Treatment in the Process of Waste Multilayer Glass Processing that Cannot be Processed by Common Technologies; Slovak University of Technology in Bratislava: Bratislava, Slovakia, 2016. [Google Scholar]
- Šooš, Ľ.; Pokusová, M.; Blecha, P.; Matúš, M.; Ondruška, J.; Čačko, V.; Bábics, J. Method of Effective Recovery of Waste Laminated Glass and Modular Construction of the Device. Slovak Patent SK8786, 8 October 2019. [Google Scholar]
- Method of Effective Recovery of Waste Laminated Glass and Modular Construction of the Device. Slovak Patent SK8786, 2 June 2020. Available online: https://patents.google.com/patent/SK8786Y1/en?oq=SK8786 (accessed on 14 April 2021).