Article Information
- Digital Object Identifier (DOI): 10.47982/cgc.8.392
- This article is part of the Challenging Glass Conference Proceedings, Volume 8, 2022, Belis, Bos & Louter (Eds.)
- Published by Challenging Glass, on behalf of the author(s), at Stichting OpenAccess Platforms
- This article is licensed under a Creative Commons Attribution 4.0 International License (CC BY 4.0)
- Copyright © 2022 with the author(s)
Authors:
- Zhufeng Pan - Shanghai Jiao Tong University
- Jian Yang - Shanghai Jiao Tong University
- Xing-er Wang - Shanghai Jiao Tong University
- Yige Wang - Shanghai Jiao Tong University
- Gang Li - Henan Zhongbo Glass Co., LTD
- Xianfang Jiang - Henan Zhongbo Glass Co., LTD
Abstract
Glass material has been widely used in modern architecture. Scratch-induced surface damage of aged monolithic glass panel leads to the strength degradation of material and thus threatens the glass safety. Therefore, in order to accurately evaluate the strength of aged glass elements, it is crucial to extract key damage features including the damage location and depth in a precise way. This study aims to develop a non-contact stage-wise scanning method to extract 3D damage characteristics on glass surface, which can further facilitate the investigation into the associated influences on the flexural strength of glass.
Coaxial double ring tests on annealed glass specimens under various magnitudes of applied loads were performed, which aimed to explore the influence of the surface damage on the flexural strength. Monocular microscope equipped with an industrial camera was used to detect damage area throughout the glass panel in the first stage rapidly. It was then followed by a chromaticconfocal scanner to precisely measure the damage depth within local damage area. The results via confocal microscope scanning were considered as the reference values. It shows that the proposed method can be a potentially alternative solution instead of confocal microscope for damage quantification.
1. Introduction
Glass is a popular building material due to its excellent transparency and mechanical performance. Although the compressive strength of glass material can be ten times higher than that of the concrete, the brittle failure of architectural glass elements can lead to severe losses (Xing-er Wang et al. 2017; Zhao et al. 2019). The surface condition of the architectural glass elements is a critical factor influencingthe mechanical strength. For as-received glass, its strength is governed by the microscopic flaws on glass surface, which is problematic in shapes and sizes (Haldimann et al. 2008).
In the design process, surface condition is determined by using the statistical theory such as microscopic flaw-based model (Kinsella and Serrano 2021) or macroscopic glass failure prediction model (Beason and Morgan 1984). Although these models are quite representative and effective, they cannot be utilized to the residual strength assessment of aged glass products, as the failure depends on several critical deep surface damage. As a result, the mechanical performance can be overestimated by the statistical model.
Scratch-induced damage is frequently seen in aged architectural glass elements such as glass facadepanels, since they are designed to be exposed to the external environment (Nategh et al. 2021; Le Houérou et al. 2003). The damage can be due to the natural aging condition such as wear, wind-borne debris, sand particles impact or vandalism damage (Schneider et al. 2012). Surface damage can cause a significant strength degradation of the material (Zhen Wang et al. 2020). Therefore, the characterization of the scratch-induced damage on the glass surface is an essential task for further assessing the safety condition of aged glass elements. In order to determine the influence of scratch-induced damage towards the mechanical strength of glass material, most study use a spherical or cone diamond indenter to generate artificial deep-to-close damage (Datsiou and Overend, 2017; Bandyopadhyay et al., 2012).
Equipment such as optical microscope is used to extract the featured information of scratch damage. However, most equipment can only obtain information such as width and location (Wiederhorn, 1969; Shand, 1965). As the strength of glass material is determined by both the shape and the depth of surface damage (Glaesemann et al., 1987), 3D characterization herein has drawn the attention of researchers (Swab et al., 2013).
To precisely measure the distance between two target planes to obtain depth information,triangulation measurement method is a classic approach, which uses the reflection of the laser to achieve the goal. La ser devices are able to conduct large-scale measurement through scanning in most scenarios. However, the precision of the laser scanners is limited to millimetre so that it cannot accurately quantify the depth of damage.
Moreover, glass is a transparent material, hence its reflection rate on the surface is too low for a laser scanner to receive the signal. In order to solve the problem, confocal microscope is considered to be a desirable solution. Researchers have applied confocal microscope to effectively determine the damage depth of different types of glass products and obtained reliable results (Datsiou and Overend 2017). However, it can only be used in measuring a limited size of specimens in the laboratory, hence it is not suitable for practical use on large-size glass panels.
In this study, a flexible method was proposed for the 3D characterization of scratch-induced damage by non-contact approach. Through a two-way sliding rail, the chromatic confocal scanner was used to smoothly measure the depth of surface damage on monolithic glass panels. The associated influence on the mechanical performance of monolithic glass panels was firstly determined by flexural strength test. The performance of the proposed system was then evaluated by the field test of glass panel.
2. Materials and methods
2.1. Glass Specimens
In this study, soda lime silica (SLS) glass specimens were 6 mm thick with a size of 100 ×100mm. Due to the convenient fabricate process, square panels were selected. All the SLS glass specimens wereannealed glass provided by the same supplier. Air side and tin side of each specimen weredistinguished by the UV light inspection. Surface damage was introduced by a scratch testing machine, as Fig.1 illustrates.
It can generate deep-to-close artificial scratch-induced damage by adding differentscratching load with a constant moving speed of 5 mm/s. Indenter is spherical shape with a diamondtip which has an angle of 120 and a radius of 0.1 mm. The applied load was determined to be 5, 10, 20 and 30N, respectively. All scratches were 10 mm long and were placed on the tin side of the specimen which was normally exposed to the external environment and was more likely to have critical surface damage.
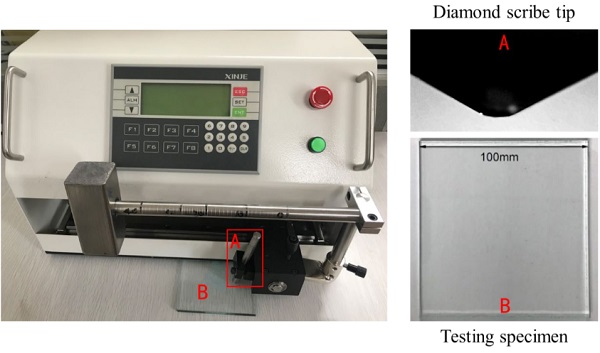
2.2. Coaxial double ring tests
Coaxial double ring (CDR) test was applied to determine the flexural strength of both intact and scratched glass specimens based on the EN1288-5, as shown in Fig. 2. Comparing with the traditional four-point bending test, CDR test can avoid edge failure by applying the load using a loading ring and a reaction ring, with the diameter of 18 and 90 mm in this test, respectively. The highest bending stress locates on the centre of the glass plate (Pisano et al. 2021). The test setup is able to determine the flexural strength of the architectural glass elements under multi-axial stress as it is close to the state under service stage (Castori and Speranzini 2019; Pisano and Carfagni 2016).
Tests for all type of specimens under scratching load were conducted on the universal testing machine with a 10 kN load cell, as shown in Fig. 3. A 0.06mm thick self-adhesive film was used to hold the fragments together for further evaluation of fracture initiation and fracture pattern after failure. The displacement rate of the test was 0.006 mm/s, corresponding to a fixed stress rate of 2 MPa/s. Ten specimens for each series were tested. Load and displacement data were recorded during the CDR tests.
The flexural strength of the glass plate can then be calculated according to EN1288-1 based on the Kirchhoff-Love plate theory as the following equation shows:
where, σf is the failure stress, h is the thickness of glass specimen, F is the load at failure, μ=0.23 is the Poisson’s ratio. R₁ and R₂ are the radius of the loading and reaction ring, respectively.3Rrepresents the radius of the circular specimen. For square specimen, R₃ can be calculated from Equation 2, where L is the edge length of the specimen:
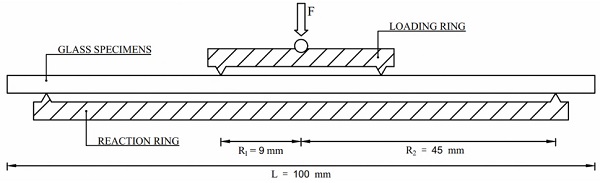
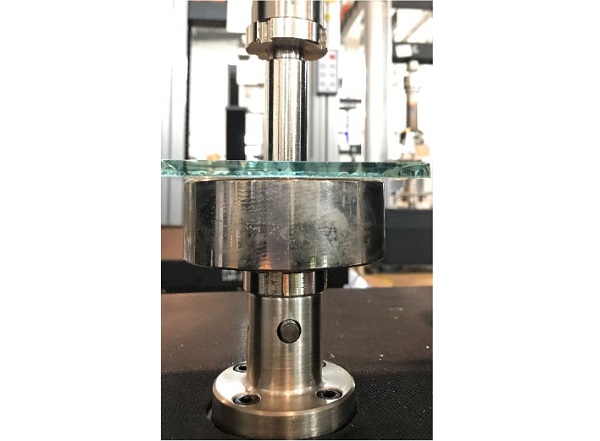
2.3. Scanning system
To achieve the goal of automatic operation and 3D characterization of the surface damage for the potential size of monolithic glass panels, a non-contact scanning system was developed. It consists of three parts, as shown in Fig. 4. A platform with the size of 1000 × 1000 mm was placed on the centre, so as to meet the demand of scratch damage detection. A two-way sliding rail was installed on the top of the platform to ensure that the scanning devices can move across the whole glass panel from side to side. Both monocular microscope and chromatic confocal scanner were fixed on the sliding rail to move in the plane automatically based on the control signal. Monocular microscope has been used to obtain the spatial information of scratch on the surface through an initial detection, see (Pan et al. 2020) into details.
Once the damage area was determined, the microscope was then replaced by a chromatic confocal scanner for a detailed scanning (Yongqing Wang et al. 2021). The physical principle of the chromatic confocal scanner is mainly from the wavelength dependence of a longitudinal chromatic aberration and the analysis of the spectral components from the reflection of light (Miks et al. 2010; Yu et al. 2018). The technical specializations of the device are listed in Table 1. The depth results can be obtained from the average value of the reflection components inside the light spot. By using the lightweight scanner, this system is able to capture the depth information of damage to meet the requirement for non-contact 3D characterization on-site replacing the confocal microscope.
Table 1: Technical specifications of chromatic confocal scanner.
A serpentine-type scanning path was set on the controller to scan through various cross sections of the damage area, as shown in Fig. 5. In order to obtain enough measured data to guarantee the reliability of the results, a least number of ten cross sections were scanned for each scratch. Each scanning cross sections were at equal distance.
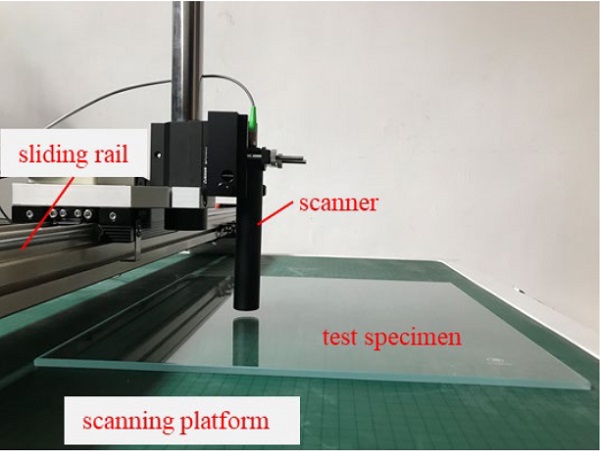
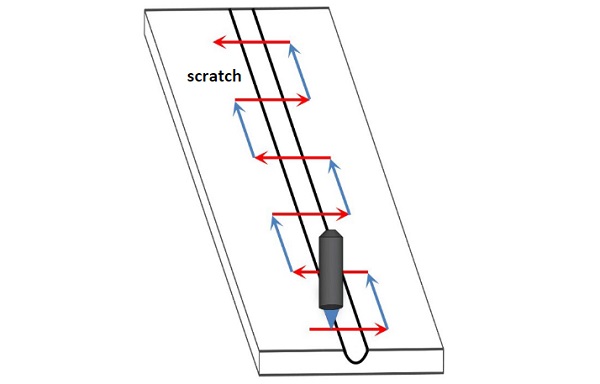
3. Results and discussion
3.1. Microscopy evaluation
Microscopic evaluation was firstly conducted on the confocal microscope to obtain the reference 3Dprofile of the surface scratch, the plan view of the surface scratch was shown in Fig. 6. It can be observed that when the scratching load is relatively low, the median cracks along the thickness of the glass is the major type of crack. With the increase of the scratching load, lateral cracks along the depth and the radial cracks on the surface are generated, whilst the interaction of lateral cracks coalesces into chips.
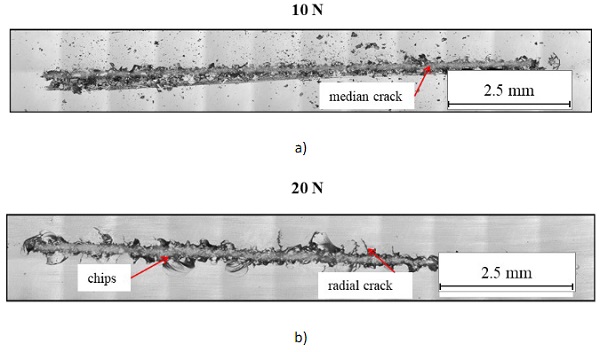
3.2. Flexural strength of glass specimens
Test results were listed in Table 2. The flexural strength of test specimens for CDR test were calculated and shown in Fig. 7. The results show that when the surface scratch was introduced, the flexural strength of glass specimens see a relatively large decrease by almost 75% the strength of the intact glass specimens even with very low scratching load. By contrast, with the increase of the load, the decreasing trend is not obvious. The results reveal that the depth of the damage has a certain upper limit. Fracture pattern for both types of annealed glass specimens is shown is Fig. 8.
The initial facture of the intact specimens is inside the loading ring, which is caused by the microscopic flaw on the glass specimen. However, the fracture of the scratched specimens occurs in the area along the scratch and directly propagates to the side at a certain angle. The fracture may indicate the requirement of surface damage characterization of aged architecture glass panel, since they are likely to contain scratch-induced damage. It acts as the main cause towards the brittle failure of the glass panel.
Table 2: Test results of coaxial double ring test.
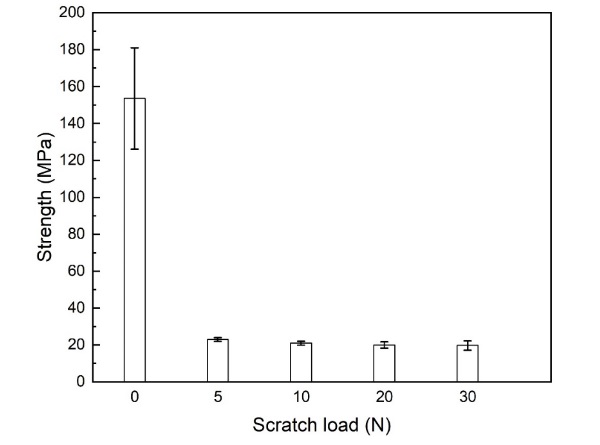
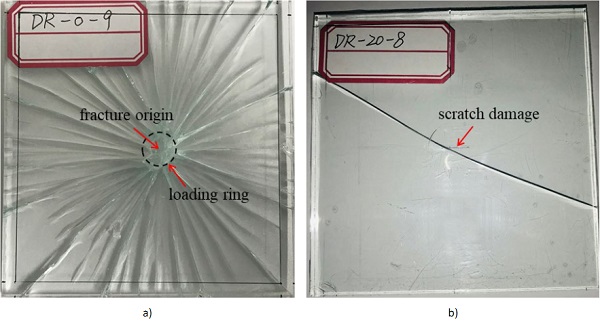
3.3. Performance of the scanning system
To assess the performance of the proposed scanning system, depth information collected from the chromatic confocal scanner were compared with the reference data obtained from the confocal microscope. The maximum scratch depth profile obtained from different cross sections of the test specimens for both devices are shown in Fig. 9. The depth profile shows a gradual increase with the rising scratch load. However, there is an upper limit, which agrees with the observations from (Haldimann et al. 2008). The chromatic confocal scanner can achieve stable performance under low scratching load. As for higher scratching load scenario, the depth profile varies due to the propagation of the median crack on different areas, whereas the deviation between both devices is relatively low so that the reliability can be guaranteed.
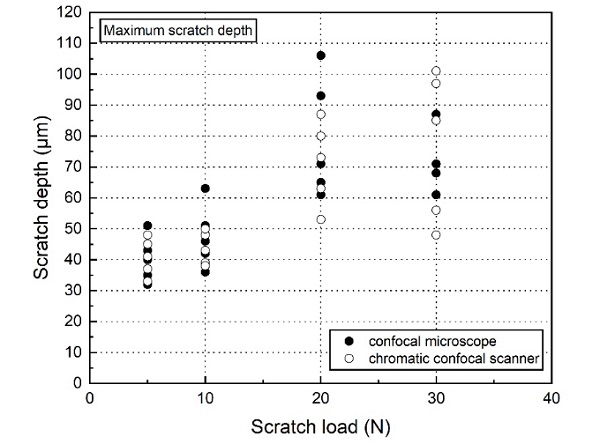
Conclusions
In this study, a non-contact method was proposed for the 3D characterization of scratch-induced surface damage for aged monolithic glass panel. CDR tests were conducted to investigate the influence of scratch-induced damage on the flexural strength of annealed glass plate. An automatic scanning system was devised to obtain 3D information especially for the depth profile of surface scratch. The performance of the proposed method was evaluated through comparative tests. Several key findings are concluded from the study:
- Comparing with the reference results by confocal microscope, the proposed system can achievestable performance with more flexibility in practical use in terms of determining critical damage in large-size architectural panels. It offers an alternative way of damage quantification for aged architectural glass panel for on-site tests.
- Damage depth on the surface of a glass panel is related to the applied load and presents an upper limit, which is validated by the flexural strength data of the glass specimens using the coaxial double ring test.
- Damage initiation of each intact specimen is different due to the microscopic surface flaws, however, all the damaged specimens failed at the location of surface scratch with severe strength reduction.
Conflict of interest
On behalf of all authors, the corresponding author states that there is no conflict of interest.
Acknowledgements
This work was supported by the National Natural Science Foundation of China [Grant No. 52078293, 51908352] and the Science Research Plan of Shanghai Municipal Science and Technology Committee [Grant No. 20dz1201301].
References
Bandyopadhyay, P., Dey, A., Roy, S., & Mukhopadhyay, A. K.: Effect of load in scratch experiments on soda lime silica glass. Journal of non-crystalline solids, 358(8), 1091-1103. (2012)
Beason, W. L., & Morgan, J. R.: Glass failure prediction model. Journal of Structural Engineering, 110(2), 197-212. (1984)
Castori, G., & Speranzini, E.: Fracture strength prediction of float glass: The coaxial double ring test method. Constructionand Building Materials, 225, 1064-1076. (2019)
Datsiou, K. C., & Overend, M.: Artificial ageing of glass with sand abrasion. Construction and Building Materials, 142, 536-551. doi:https://doi.org/10.1016/j.conbuildmat.2017.03.094. (2017)
Glaesemann, G. S., Jakus, K., & Ritter Jr, J. E.: Strength variability of indented soda‐lime glass. Journal of the American Ceramic Society, 70(6), 441-444. (1987)
Haldimann, M., Luible, A., & Overend, M. Structural use of glass. IABSE. (2008)
Kinsella, D., & Serrano, E.: Failure modelling of glass plates in biaxial loading: using flaw-size based weakest-link systems. Glass Structures & Engineering, 6(4), 397-424. (2021)
Le Houérou, V., Sangleboeuf, J. C., Dériano, S., Rouxel, T., & Duisit, G.: Surface damage of soda–lime–silica glasses: indentation scratch behavior. Journal of Non-Crystalline Solids, 316(1), 54-63. (2003)
Miks, A., Novak, J., & Novak, P.: Analysis of method for measuring thickness of plane-parallel plates and lenses using chromatic confocal sensor. Applied optics, 49(17), 3259-3264. (2010)
Nategh, S., Missinne, J., Vijverman, P., Van Steenberge, G., & Belis, J.: Effect of ultrashort laser-induced surface flaws on architectural glass strength. Construction and Building Materials, 295, 123590. (2021)
Pan, Z., Yang, J., Wang, X.-e., Liu, J., & Li, J.: Surface scratch detection of monolithic glass panel using deep learning techniques' International Conference on Computing in Civil and Building Engineering. pp. 133-143. (2020)
Pisano, G., Bonati, A., & Royer-Carfagni, G.: The effect of size and stress state on the strength of architectural glass. Experiments versus theory. Construction and Building Materials, 283, 122635. (2021)
Pisano, G., & Carfagni, G. R.: Towards a new standardized configuration for the coaxial double test for float glass. Engineering Structures, 119, 149-163. (2016)
Schneider, J., Schula, S., & Weinhold, W.: Characterisation of the scratch resistance of annealed and tempered architectural glass. Thin Solid Films, 520(12), 4190-4198. (2012)
Shand, E. B.: Strength of glass—the Griffith method revised. Journal of the American Ceramic Society, 48(1), 43-49. (1965)
Swab, J., Thies, S., Wright, J., Schoenstein, J., & Patel, P.: Influence of surface scratches on the flexure strength of soda-lime silicate and borosilicate glass. Experimental Mechanics, 53(1), 91-96. (2013)
Wang, X.-e., Yang, J., Liu, Q.-f., Zhang, Y.-m., & Zhao, C.: A comparative study of numerical modelling techniques for the fracture of brittle materials with specific reference to glass. Engineering Structures, 152, 493-505. (2017)
Wang, Y., Xi, M., Liu, H., Ding, Z., Du, W., Meng, X., et al.: On-machine noncontact scanning of high-gradient freeform surface using chromatic confocal probe on diamond turning machine. Optics & Laser Technology, 134, 106569. (2021)
Wang, Z., Guan, T., Ren, T., Wang, H., Suo, T., Li, Y., et al.: Effect of normal scratch load and HF etching on the mechanical behavior of annealed and chemically strengthened aluminosilicate glass. Ceramics International, 46(4), 4813-4823. (2020)
Wiederhorn, S. M.: Fracture surface energy of glass. Journal of the American Ceramic Society, 52(2), 99-105. (1969)
Yu, Q., Zhang, K., Cui, C., Zhou, R., Cheng, F., Ye, R., et al.: Method of thickness measurement for transparent specimens with chromatic confocal microscopy. Applied Optics, 57(33), 9722-9728. (2018)
Zhao, C., Yang, J., Wang, X.-e., & Azim, I.: Experimental investigation into the post-breakage performance of pre-cracked laminated glass plates. Construction and Building Materials, 224, 996-1006. (2019)