Authors: Helenice Maria Sacht, Luís Bragança, Manuela Almeida and Rosana Caram
Source: Sustainability 2021, 13(10), 5437, MDPI
DOI: https://doi.org/10.3390/su13105437
Abstract
The correct specification of glazings for façades can reduce the energy consumption in buildings. The heat exchange occurs through transparent surfaces and radiation reaches the building as light and heat. Therefore, glazings significantly contribute to the heat transfer between outdoor and indoor spaces and act directly on daylighting and thermal comfort. This paper reports on the spectrophotometric characterization of glazings transmittance for the study of components of a modular façade system and its suitability for the climate of Portugal (temperate climate). The study focused on results of spectrophotometric measurements of optical properties, specifically the transmittance of some types of glazings (solar control, self-cleaning, low-e, float, and extra-clear) and two types of double glazings. The results show the percentage of transmission to ultraviolet, visible, and near-infrared regions and its importance, which enabled the analysis of the glazing efficiency regarding daylighting and the correlation to thermal performance. Subsidies and indications for the specification and adequate uses of transparent surfaces have been presented and complemented the datasheets available from the manufactures.
1. Introduction
A large variety of transparent materials is available for use in buildings façades. However, all glazing properties must be considered in the choice of material. The selection of glazings should be a careful process of evaluation and weighing of tradeoffs. Among the characteristics required for the specification of transparent materials, the spectrophotometric behavior is an important factor to be considered, as it enables improvements in the thermal and visual comfort of a building.
Many studies have been carried out in this respect, both for glass and other transparent materials, mainly focused on penetration of UV rays through the glazings. In order to curb excessive penetration of UV rays, optically functional glasses have been proposed and the performance assessments of the glazed materials have been measured and reported by Kim et al. [1]. Results have shown that UV protection glass is more effective in controlling the UV rays of natural light; clear glass treated with a UV protection film would provide excellent control of UV penetration and a pair of clear and UV protection glass sheets treated with a UV protection film should be recommended; this achieves 96.7% UV protection performance from natural light.
Goia et al. [2] investigated the spectral and angular behavior of different PCM glazing samples, characterized by different thicknesses of PCMs, by means of a commercial spectrophotometer, and by means of a dedicated optical testbed that includes a large integrating sphere with a diameter of 0.75 m. The experiments have pointed out the highly diffusive behavior of the PCM layer, with increasing weight of the direct-to-diffuse transmission mode as the PCM layer thickness increases.
Morettia et al. [3] studied the thermal and optical characterization of three polycarbonate systems for buildings (with different numbers of chambers and geometry). Thermal transmittance measurements were carried out using a hot-box apparatus, in order to assess the impact of the connection joints on the effective U-value and to compare it with data declared by manufacturers, usually referred to as the center of the component. The investigated polycarbonate systems could be a valid solution in place of classic windows in commercial buildings and the study provides deepened knowledge about the polycarbonate panels’ thermal and optical behavior and a set of useful data for accurate analyses in building integration.
Baldinelli [4] presented the spectral data on the wavelength field of interest for solar radiation, showing the high transparency levels of glazing systems made by an internal layer (stratified glass, air gap, float glass) and external layer (stratified glass), as well as the good reflective properties of the aluminum in the shading system. According to the same author, glazing optical properties depend on the incident angle between the surface and the ray direction: as this angle deviates from the normal direction (0°), transmissivity decreases, reflectivity increases, and absorptivity increases. The variation of optical properties with the incidence angle depends on glass type and thickness; in particular, it is more pronounced for multiple-pane glazing systems.
Berardi [5] points out that with recent advances in glazing technology, manufacturers can control how glazing behaves in the different portions of the spectrum. Coatings may control the passage of long-wave solar radiation through transmission and/or reflection. In the past, coatings on windows that reduced the solar gain also reduced the visible transmittance and were hardly accepted by occupants. However, new high-performance tinted glass and low solar-gain (or low-E) coatings make it possible to reduce the solar heat gain with little to no reduction in the visible transmittance. A low-E coated glass has a microscopically thin transparent metal coating that reflects longwave infrared energy during winter and blocks external shortwave gains during summer.
Despite there being studies on the determination of optical properties of glazing and other materials for façades, with the development of new materials, further studies are needed for different types of glass, as well as considering the behavior of double glazings and analyzing the relationship between those properties with thermal and daylighting behavior.
1.1. Glazings and Solar Spectrum
People typically spend many hours in buildings bathed in the ultraviolet, visible, and infrared radiation produced by natural or electric lighting. This radiation can damage tissue regardless of whether or not it affects the visual and circadian systems [6]
The radiation transmission through glazings depends mainly on factors, such as angle of radiation incidence, thickness, chemical composition, and superficial characteristics of the glazing. The angle of radiation incidence is the angle between the direction of the radiation and the normal one (90~) to the surface under analysis [7]. The transmission of glazings also depends on the wavelength of the incident radiation. The glazing acts as an opaque material for certain regions of the spectrum, for example, it exhibits opaque behavior and prevents the crossing of radiation for wavelengths shorter than 300 nm and longer than 5000 nm [8].
The main thermal exchanges, as well as the passage of light into the interior of a building, occur on translucent or transparent surfaces. Therefore, such surfaces act directly on thermal comfort and daylighting. Regarding transparent glazing, part of the energy from the solar radiation (Figure 1a) is directly transmitted to the interior of the environment, which distinguishes opaque surfaces from translucent ones. Reflection, absorption, and transmission also occur on translucent surfaces (Figure 1b), however, the transmission takes place in a different way. The light propagates when it penetrates translucent glazing and leaves it as a diffuse light, and not as a direct one.
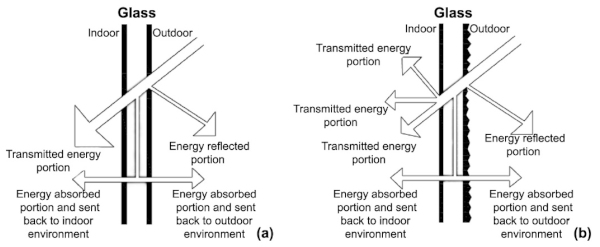
The behavior of transparent materials when they receive solar radiation is to react selectively, that is, the amount of energy they absorb, reflect, or transmit depends on the wavelength of the incident ray. When the angle of incidence is not normal to the plane and is increased, the reflected energy becomes more and more, while the transmitted and absorbed energy decreases, although the relationship that corresponds to the normal incidence approximately remains the same between them.
The total value of the radiation transmitted, reflected, and absorbed by glazings is an important variable for the calculation of the solar heat gain coefficient, which depends on the optical properties of the material, solar factor, incident solar radiation, overall heat transfer coefficient (U factor), and difference of temperature between the outdoor and indoor environments.
The visible spectrum is the most known solar radiation. However, two other important bands are ultraviolet (UV) and infrared (IV). Figure 2 shows the solar radiation incidence divided by spectra. Although the sun emits radiation in an extensive band of electromagnetic waves, only a narrow band of the solar spectrum is perceptible. Wavelengths longer than 1500 nm reach the terrestrial surface in small proportions.
![Figure 2. Solar Spectrum [9].](/sites/default/files/inline-images/Fig2_315.jpg)
For the use of solar energy, the only radiation considered is the one whose wavelength ranges between 290 and 1800 nm, or, more specifically, up to 1500 nm [10], as wavelengths longer than those reach the terrestrial surface in a very reduced way and are absorbed by the water vapor and carbon dioxide present in the atmosphere. Wavelengths shorter than 290 nm are absorbed by the ozone layer [7].
The solar spectrum is divided into the ultraviolet UV region (100 to 380 nm), visible region (380 to 780), and infrared region (780 to 3000). The transmission of the glazings for each interval influences some characteristics.
1.1.1. Ultraviolet (UV) (100–380 nm)
The ultraviolet band is more energetic than the light (it has a shorter wavelength), therefore, it penetrates the skin more deeply and causes burns depending on the time of exposition to the solar radiation. Although only 1 to 5% of the ultraviolet radiation reaches the terrestrial surface, it must not be ignored due to the effects it exerts.
In some situations, such radiation may not be important, as in recovery areas in hospitals, as it is responsible for the synthesis of Vitamin D through the skin and exerts a bactericide effect. However, it compromises the durability of materials by the discoloration of the tissues, as it has photochemical capacity. The ultraviolet region that causes the discoloration of the materials is between 315 and 380 nm. Such radiation is also responsible for tanning.
The ultraviolet radiation is subdivided into three intervals: UV-C (between 100 and 280), UV-B (between 280 and 320), and UV-A (between 320 and 380). The UV-A radiation (between 320 and 380) causes the direct tanning of the skin with weak erythema or sun-burn. The maximum reaction of the erythema occurs 72 h after the exposition to the sun. The continuous exposition causes not only fast aging of the skin but also carcinogenic implications. The UV-B radiation causes an erythema response and, in association, indirect tanning. Under intense and frequent radiation, UV-B may produce skin carcinomas. In such a case, the maximum reaction occurs between 6–20 h after exposition. UV-C radiation does not reach the Earth, as wavelengths below 290 nm are absorbed by the ozone layer of the atmosphere. Such radiation is a germicide and highly harmful to the human skin, due to its high energy content [11].
1.1.2. Visible (Vis) (380–780 nm)
The visible region is associated with the intensity of the white light transmitted and influences directly the degree of daylight of an environment. It is also called luminous and enables the visual sensation of days passing. It is the visible portion of the incident solar radiation in the direction normal to the surface plane.
The visible light carries enough energy to stimulate chemical reactions in the eyes and the functioning of the visual system. This wavelength follows the sequence: violet, blue, green, yellow, orange, and red, however, the human visual system is more effective in the wavelength that corresponds to green and yellow. Besides being fundamental to humans, the visual system is indispensable for the development of vegetables, because of the photosynthesis process, which requires visible light. Chlorophyll, the agent responsible for the vegetable cellular respiratory process, absorbs specific regions of the spectra.
1.1.3. Infrared IR (780–3000 nm)
The infrared region is invisible to the visual system, although it is noticed in the form of heat. It interferes with the indoor conditions of the environment through solar heat gain, therefore, it cannot be ignored. It is divided into three bands: short-wave infrared, or near-infrared, whose wavelengths range between 780 and 1400 nm, mid-wavelength infrared, which ranges from 1400 to 3000 nm, and, finally, long-wavelength infrared, which includes the radiation that results from heated bodies, whose wavelengths are longer than 5000 nm.
The near-infrared corresponds to the large part of the solar spectrum that crosses a colorless glass, for instance, in high proportions. References of some specifiers regarding such a region of the spectrum include statements as “glass is generally opaque to the infrared”, however, this is wrong information [8].
A spectrophotometric characterization of glazings was accomplished for the study of components for a modular façade system in Portugal. The study focused on results of spectrophotometric measurements of optical properties, specifically, the transmittance of some types of simple glazings and two types of double glazings [12,13,14].
The results enabled the verification of the glazing efficiency in terms of daylight and the correlation to thermal performance and provided subsidies and indications for the specification and adequate uses of transparent surfaces. They also complemented the datasheets of the manufactures. An evaluation of the transmission of glazings by spectrophotometric tests enables the analysis of the different regions of the spectrum (ultraviolet, visible, and infrared) individually and not as a whole, as it occurs with parameters, such as solar factor and shading coefficient, which are calculated in a global way without taking into account the differences of behavior per region of the spectrum.
2. Materials and Methods
The development of this research included the following tasks: I. Characterization of glazing materials; II. Preparation of the spectrophotometer; III. Cleaning, Identification, and Fixation of the Specimen on the Device; and IV. Description of the Spectrophotometric Tests.
2.1. Characterization of the Glazing Materials
Samples of 6 mm thick glazings were used in the spectrophotometric tests. Ten types of simple glazings and two types of double glazings were analyzed. The double glazings were mounted on hard paper with the aid of a device (Figure 3a,b).
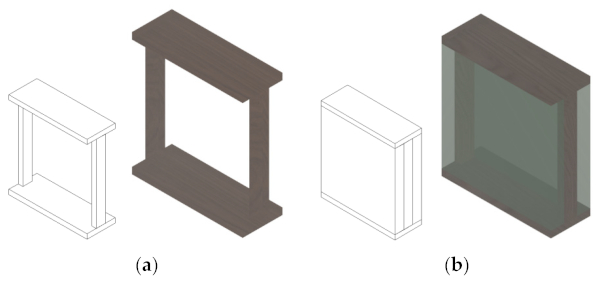
This device enabled the configuration of the double glazings with an indoor air layer. The samples were placed 6 mm from each other in the spectrophotometer, differently from the usual position, that is, 12 mm. However, the results would be significant for the analysis of the influence of the air layer on the transmission of the double glazings.
Rectangular samples of 50 mm × 50 mm were used for all eight types of glazings. The interval of the spectrum was 200 to 1100 nm, which comprised three regions: ultraviolet (200 to 380 nm), visible (380 to 780 nm), and near-infrared (780 to 1100 nm). Table 1 shows the characteristics of the samples analyzed.
Table 1. Characteristics of the Glass Specimens.
The glazings were selected mainly according to their characteristics regarding overall heat transfer (U-value) and Visible Transmittance. The requisite of thermal quality is the U-value, to which acceptable maximum values (Umáx) are limited and reference values (Uref) are recommended, according to RCCTE Portuguese Code [15]. The maximum superficial U-values (Umáx) acceptable in a current zone and the reference values (Uref) for the elements of the buildings envelope are shown in Table 2.
Table 2. Maximum surface heat transfer coefficient acceptable and reference surface heat transfer coefficient [15].
Therefore, the U-value of the glazings should not exceed 3.30 W/m2·°C, so as to meet most zones. Moreover, maximum solar factors acceptable (g┴) are recommended. No glazing of any building that is not northern-oriented (between the northwest and the northeast) and whose total area does not exceed 5% of the useful pavement must show a solar factor corresponding to the glazed void with the respective protection devices 100% active that exceeds the values indicated (Table 3).
Table 3. Maximum Solar Factor accepted for glazings [15].
Table 4 [16] shows other characteristics of glasses, as the U-values, solar factor, shading coefficient, and relative heat gain of the glazings studied. Regarding the spectrophotometric tests, images of the samples will be shown, so as to provide the visual aspect of the selected material. The studied glasses are:
Table 4. Characteristics of the Glazings.
- Glass A is a glass with a solar control metallic foil that confers its characteristics of solar control;
- Glass B is a green glass with a solar control metallic foil that confers its characteristics of solar control;
- Glass C is self-cleaning glass manufactured by depositing a transparent layer of photocatalytic and hydrophilic mineral material onto clear glass;
- Glass D is a glass with a low-emissivity foil;
- Glass E is a glass with an extremely low-emissivity foil;
- Glass F is clear float glass that has been coated with metallic oxides by magnetically enhanced cathodic sputtering under vacuum conditions and solar control properties;
- Glass G is a high quality, clear annealed glass, manufactured by the float process;
- Glass H is a highly transparent extra clear glass, which has very little residual color;
- Glazings 04 and 07 are double glazings.
Table 5 shows the samples of the selected glazings. The glazings with a metallic-origin coating, mainly Glass D, Glass E, and Glass F oxidize in contact with the air. Therefore, in the compositions of double glazing, the coating must be placed inside. In the samples, the rusty part corresponds to the abrasion band that shows the face with the film, so that the glazings could be correctly positioned in the spectrophotometric characterization tests.
Table 5. Specimens of Glasses.
2.2. Preparation of the Spectrophotometer
According to the ASTM [17], the spectrophotometer is the ideal equipment to provide the data of transmission percentage to the ultraviolet, visible, and near-infrared regions. It also enables a sweeping in the spectrum only in the region of interest.
A spectrophotometer was used in the tests. It enables a monochromatic light sheaf to pass through a sample and measures the amount of light absorbed (Figure 4). With the aid of a prism, it separates the light into sheaves of different wavelengths (as in the rainbow, with the separation of the colors of white light. Therefore, a monochromatic light sheaf (of only one, or almost only one wavelength) can pass through the sample. The spectrophotometer shows the amount of light absorbed at each wavelength and provides data on absorption, reflection, and transmission of the material for different incidence angles.
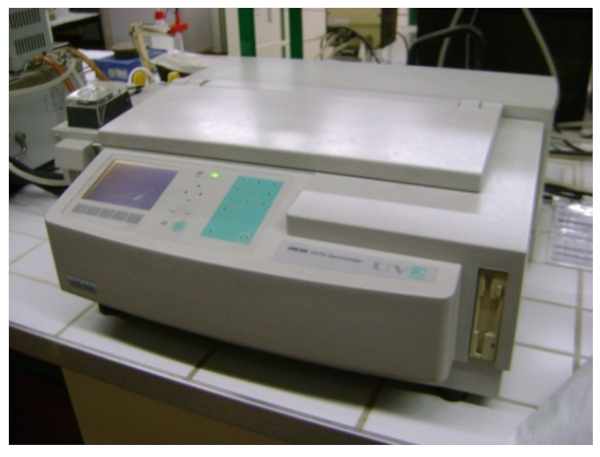
UNICAM UV/VIS was the device used for the tests. It provides data on absorption, reflection, and transmission of the tested materials. By virtue of our objective, only the transmission mode was used. A tungsten lamp was used for the whole spectrum. The samples were tested at 0° with the normal incidence (sheaf perpendicular to the sample) and the sheaf incidence was in accordance with the coating of the samples, as it followed the recommendations of the manufacturer for both simple and double glazings analyzed.
In the specific case of transmission, graphs can be generated with the transmission percentage for the ultraviolet, visible, and near-infrared regions and the results can be compared among themselves or with a colorless simple glazing, for example, for the verification of the efficiency of the glazings regarding the illuminance level [7].
The information of the glass manufacturers is limited to values of mechanical resistance, acoustic insulation, and energetic transmission of solar radiation. The latter is treated in a generalized way and the data on the visible region and solar factor ignore the behavior of the material in the ultraviolet and infrared radiation. The data usually regard the transmittance for the incidence of normal radiation to the surface, which occurs in a minimum period of time. Some information refers to the solar heat gain coefficient. However, in most cases, no data on the infrared are provided, which hampers an individual and correct analysis of the spectral behavior of the glass.
The highest concentration of infrared is in the 780 to 1500 nm interval and the percentage for wavelengths longer than 1500 nm is very small. Therefore, analyses for the 780 to 1100 nm interval used in our research are valid. This is the limiting interval in which the device utilized performs the sweeping
The data provided by the spectrophotometer enabled the creation of curves with the transmission data and analysis of the integration of both areas that correspond to each region of the spectrum (ultraviolet, visible, and infrared) and the total area of the curves. Therefore, the transmission could be compared in characteristic intervals.
2.3. Cleaning, Identification, and Fixation of the Specimen on the Device
Prior to the tests, the samples were cleaned with acetone and paper. No water was utilized, as the ferrous minerals might react with the films of some glazings and damage them. The samples were identified by a permanent marker in their thickness, as the main faces could not be obstructed. After the cleaning and identification, the samples were stored in a plastic container and covered with aluminum foil so that no dirt would be accumulated until the beginning of the tests.
The specimens were carefully fixed on the device with adhesive tape so that the test would not be damaged and the samples would not be at an incline regarding the incidence of the sheaf or the sheaf would not focus on the adhesive tape. The samples of double glazing were mounted with the aid of a hard paper device and fixed with adhesive tape.
2.4. Description of Spectrophotometric Tests
The spectrophotometer was set at 100% transmission for calibration. A baseline was then determined so as to exclude any possible error from the equipment. After calibration, the equipment was kept open so that the sample could be placed inside it (Figure 5).
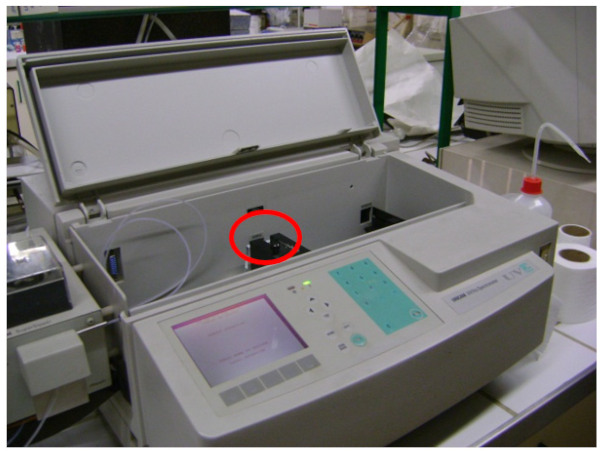
The glazings with special films (except the self-cleaning one) were tested with their face treated, facing the inner part of the device, and not in contact with the sheaf of light. Such a procedure was performed according to the manufacture’s recommendation that the face must face the inner part of the glazing composition so that the coating should be intact, as it easily oxidizes when it comes in contact with the air.
The software started the sweeping procedure at 200 nm with the equipment closed and ended at 1100 nm. A 200 to 1100 nm spectrum was analyzed in this spectrophotometric characterization test. Another division was created in this interval and other sub-intervals were generated to characterize the ultraviolet (300 to 380 nm), visible (380 to 780 nm), and part of the infrared (780 to 11 nm) regions.
The process used a graph generated with the results during the sweeping. Three samples of each type of glazing were tested for the establishment of a mean value of the transmission per total interval. The process was carefully conducted, especially in the fixing of the sample, so that no other baseline would be established. Each test was performed for 3 to 4 min (the time of both preparation and cleaning was disregarded).
The same procedures were employed in the testing of the samples of double glazings. The only difference was the assembling of the samples with the aid of adhesive tape in the hard paper device for the further fixation of the set to the spectrophotometer. The data obtained enabled the generation of graphs with the transmission curves and the integration of both the areas corresponding to each region of the spectrum analyzed and the total area of the curves, which will be provided in the results.
3. Results
The experimental results from the spectrophotometer are provided in graphs and tables for a good visualization of what occurs regarding transmittance inside the glazings studied for each sample subjected to normal incidence (90°) of radiation. Such results will be shown per type of glazing and in a graph and a table that includes the eight types of glazings analyzed.
The samples were tested in an interval that corresponds to part of the solar spectrum (100 to 1100 nm). Another sub-division was performed for the characterization of the ultraviolet-UV (200 to 380 nm), visible (380 to 780), and part of the infrared-IV (780 to 1100 nm) regions. The data enabled the generation of curves integrated to the areas of each region of the spectrum, as well as the integration of the total area of the curves so that the transmission values of the glazings could be obtained.
Regarding the transmission in the visible region, the use of glazings whose transmission ranges between 30 and 50% is recommended, as they guarantee environments of satisfactory illuminance level and enable the development of activities that require precision of the visual system. The following intervals of luminous transmission (LT) can be established as parameters [8]:
- LT < 30% weak luminous transmission;
- 30% ≤ LT ≤ 50% medium luminous transmission;
- LT > 0.50 strong luminous transmission.
The LT coefficient should be between 30 and 50% on vertical surfaces and 25% and 40% in covered ones. Besides the transmission in the visible region, which is usually the parameter provided by the manufacturers of glazings, the transmission in the intervals of the ultraviolet and infrared must also be considered. The curves and tables of results will be discussed based on such considerations and from the point of view of thermal comfort and daylighting.
3.1. Glass A and Glass B: Solar Control—Translucent and Green
The transmission of Glass A with a solar control coating in the UV is lower (9.24%) in comparison with a simple glass, such as Glass H (extra clear). The transmission in the visible region is around 47.28% and 24.52% in the infrared. The latter value indicates lower internal heating, in comparison with the glasses previously analyzed (Figure 6).
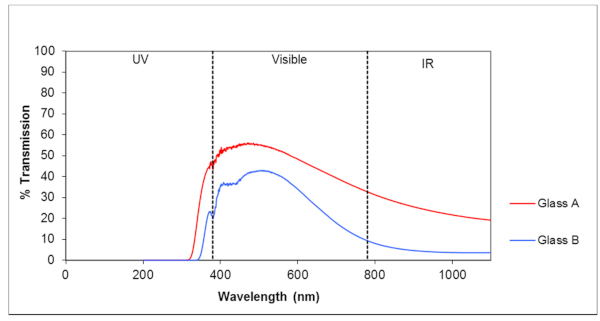
The Glass B (green solar control) showed a large transmission decrease in all regions of the spectrum, mainly in the UV and infrared regions, with percentages of 2.85% and 4.80%, respectively. Regarding visible transmission, the value was 14%. The transmission in the UV and IV regions has confirmed the solar control characteristics of the glass, which are lower in comparison with the simple float glasses. In comparison with Glass H (extra clear, highest transmission in all regions), for instance, differences of 28.8%, 58.78%, and 81.38% were found in the UV, visible, and IV regions, respectively.
3.2. Glass C: Self-Cleaning
The percentage of transmission of the Glass C (self-cleaning) in the UV region (17.03%) is a little lower than that of Glass G (clear float annealed). Regarding visible light, it transmits 82.52%, which guarantees a high transmission that favors the conditions of daylight. In terms of infrared radiation, its transmission is close to that of Glass G (70.78%), which indicates that its use will cause higher heating in the indoor environment, however with a small decrease of UV radiation (Figure 7). In this case, the self-cleaning coating does not cause large differences regarding transmission in comparison to the Glass G (clear float annealed), a float glass, which is an advantage because, besides higher daylighting and internal heating, a self-cleaning function is expected.
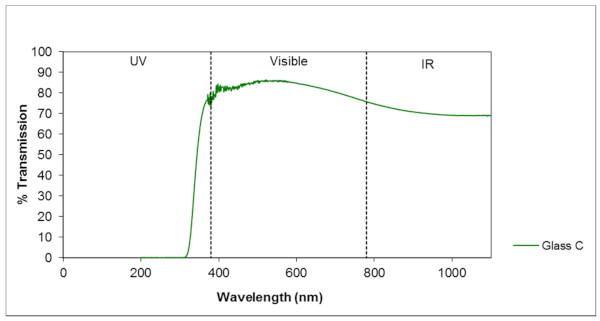
3.3. Glass D and Glass E: Low-e
The two types of glasses with a film of low emissivity led to very similar transmission values, with differences of approximately 1% in the intervals analyzed and 0.4% in the total transmission. The transmission of the Glass D (low-e) was 79.22% in the visible region, which can be considered strong and superior to that of the solar control glasses. Such glasses reduce thermal losses by radiation in the winter. The 52.75% transmission in the IV region favors heating (Figure 8).
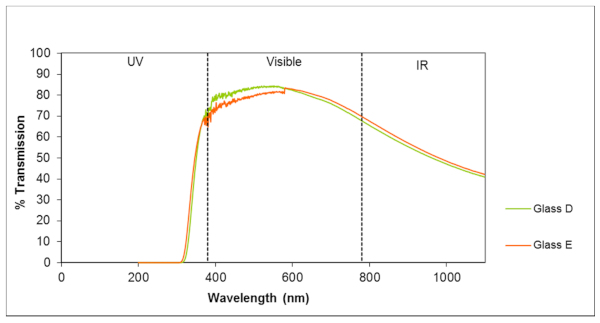
The differential of the Glass E is its emissivity inferior to that of the Glass D. However, in terms of transmission, its behavior was very similar, with 15.94% transmission in the UV region, 78.07% in the visible region, 54.38% in the IV and 57.10% total transmission. The main difference in its curves in relation to the previous ones is in the visible region, to which a 1.15% higher transmission was led.
3.4. Glass F: Solar Control
The transmission of the Glass F (clear float), solar control glass is 10.19% in the UV region, 61.56% in the visible region and 20.47% in the infrared region analyzed. Its percentage of transmission in the IV region is inferior to that of the glasses analyzed, which indicates lower heating with its used. In comparison to Glass A (solar control), for instance, it shows a 14% increase in the transmission in the visible region, which suggests better conditions of daylight (Figure 9).
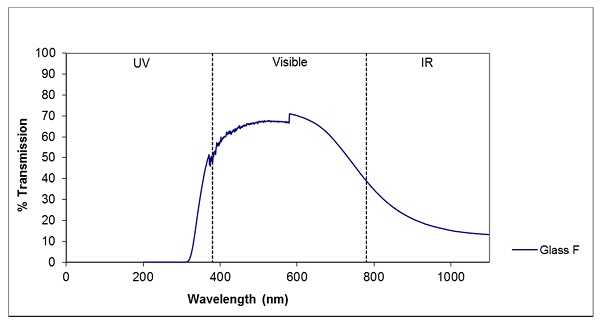
3.5. Glass G and Glass H: Simple Float
Colorless transparent materials usually exhibit similar spectrophotometric behaviors. Figure 10 shows the transmission of the Glass H (extra clear) is high in the visible region (88.92%) and in the infrared region (86.18%), which indicates a strong luminous transmission. Moreover, it favors the heating of the indoor environment, as it shows high transmission in the infrared region. Such features have confirmed its efficiency for use in strategies of direct and indirect gains, as Trombe walls and greenhouses, for instance. Its specification as extra light glass has also been confirmed by the high transmission in the visible region.
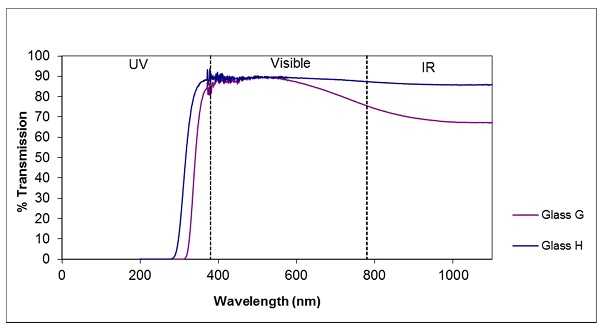
Although it is also a float glass, Glass G shows lower ultraviolet radiation transmission in comparison to Glass H (around 12%). Its transmission in the visible region is near the one of the Glass H (85.36%). However, according to the use, if the aim is a float glass of greater blockage of the wavelengths of the ultraviolet, Glass G shows a lower transmission for this interval. According to the analysis of the spectrophotometric characteristics between the Glass H and Glass G, the former has shown more adequate for use in Trombe walls.
There are some indentations in the solar spectrum due to the absorption of part of the radiant energy by some of the gases that make up the atmosphere (Figure 2). When comparing the composition of the solar spectrum with the spectrophotometric behavior of simple float glasses, it is concluded that simple float glasses are very transparent to solar radiation.
3.6. Double Glazings 04 and 07
A substantial decrease was observed in all regions of the spectrum for Glazing 04, composed of the Glass B (green solar control), an air layer and Glass E (extremely low-e). The visible and infrared regions, which showed percentages of 25.15% and 2.63%, respectively, must be highlighted. The lowest transmission values among all analyses in the infrared interval have proven the efficiency of the use of such glazing (25.15%) in the decrease of nominal cooling needs in terms of visible transmission (Figure 11). A decrease in the transmission is observed for the composition of a green glass of solar control (outer) and low-e glass (inner).
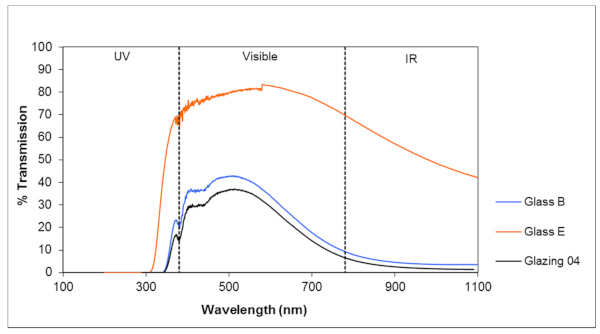
Glazing 07, composed of Glass C (self-cleaning), a layer of air, and Glass G (clear float annealed) showed the second-highest percentage of transmission in the UV region (23.92%). Regarding visible light, it transmits 70.34%, which guarantees high daylight. In terms of infrared irradiation, its transmission is close to that of low-e Glass D (low-e) and Glass E (extremely low-e), which indicates its use may heat the internal environment, however, with a small decrease in the UV radiation transmission (Figure 12). In this case, the self-cleaning coating caused no differences in terms of transmission in comparison to the composition of double glazing with the low-e glasses analyzed.
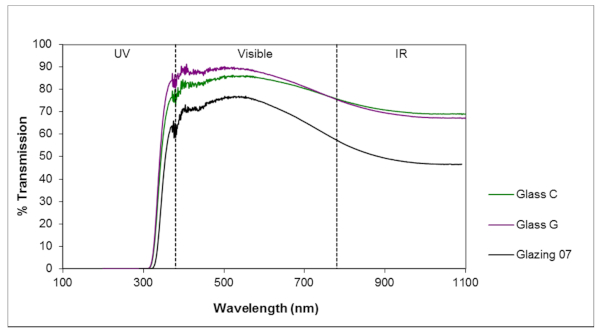
4. Discussion
Table 6 shows the transmission in the intervals analyzed: ultraviolet, visible, and infrared of each glass as well as the total transmission. The lowest and highest values are highlighted for each transmission interval and total transmission.
Table 6. Transmission of radiation for the ultraviolet, visible, and near-infrared regions.
The Glass H, extra-clear float glass led to the highest percentages of radiation transmission in comparison to the other glasses analyzed, which has confirmed its definition as an extra clear float glass and efficiency for use in systems of passive heating, as Trombe walls. The lowest values of total transmission and in the visible and infrared intervals were found for the 04 double glazing, which employs Glass B (green solar control) in the outer pane and Glass E (extremely low-e) as in the inner pane. The lowest transmission result was observed for the simple Glass B for the ultraviolet region.
Such a characteristic has proven that the performance of the green glass was good for use in climates in which the decrease in the nominal cooling needs is a priority. The results of previous studies of computational simulations of thermal performance have shown the cooling energy needs were the lowest with the use of such glass in the outer pane, joint with the inner low-e Glass E (extremely low-e) [14]. In terms of total transmission, the lowest and highest values were found for the 04 double glazing and Glass H simple glazing, respectively (Figure 13).
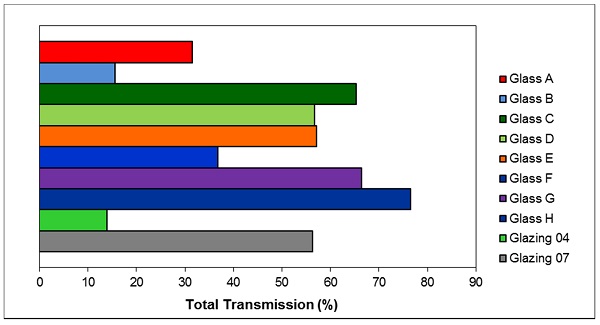
Besides the Glass H, which can be indicated for use in Trombe walls, Glass G and Glass C must be highlighted, as they have shown the highest values of total transmission in the characteristic intervals. The total transmission of glass 07 (Glass C-self-cleaning in the outer pane and Glass G-clear float annealed, in the inner pane), mainly in the infrared region, has also proven its efficiency regarding the decrease in the heat energy needs. The data of transmission of solar radiation are directly related to the thermal comfort conditions, mainly in relation to the results for the infrared interval.
Figure 14 clearly shows the maximum and minimum transmission values for Glass H (extra clear) and glazing 04, respectively. Glass B (green solar control) enables good illumination and weakens the infrared radiation in the interval analyzed, reducing the heating in this region. Green glasses are known to always tend to decrease transmission in longer wavelengths.
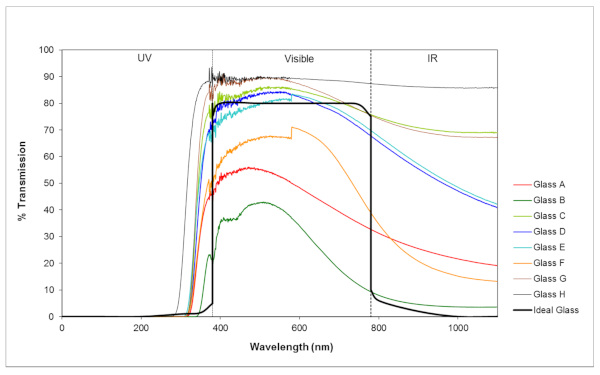
Another factor that must be highlighted is the diversity of behaviors regarding the transmission shown by the glasses (see above graph), even for materials of similar characteristics in terms of composition.
According to the results, the solar control glasses (colorless, green, Glass F-clear float-solar control and Glass A- solar control) have shown little transparency to the ultraviolet, therefore they can be adequate for shop windows, commercial centers, museums, and even residences. Glazing 04 can be highlighted in terms of control of ultraviolet solar radiation, with the lowest transmission for this region. This spectrum band has high energetic power, which deteriorates and discolors the materials and may even cause skin diseases. However, in some situations, its incidence can be important, as in recovery areas in hospitals, as it is responsible for the synthesis of some vitamins.
Glass C, which has a self-cleaning coating, exhibited good thermal behavior, based on the results of thermal performance simulations of other research stages [14]. Such results show the performance of this glass is good, regarding the decrease in the heat energy needs.
Figure 14 also shows a curve of the possible behavior of an ideal glass in terms of radiation transmission, which varies in function of the climate for which the material will be indicated. For instance, the glass considered ideal for façades in hot regions and exposed to solar radiation daily should present a good transmission in the visible region and light transmission in the infrared. The material must allow the entry of light and avoid the entry of heat from the predominant climatic conditions. As the climate in Portugal is temperate, the ideal glazing should work as a barrier against ultraviolet radiation, enable the passage of the visible light to favor daylighting, and enable a part of Infrared light to promote passive heating, thus the low-e glass use is a good indication.
Although the study was done for the climate of Portugal, it is emphasized that it can be consulted for application in other locations, according to the needs that are sought in the application of glazing suitable to the climate. For example, if an application in architecture requires minimizing issues related to the fading of surfaces, glass with the greater transmission in the ultraviolet region should be avoided. If the application objectives of the glazing are to improve the conditions of daylight, greater values of transmittance should be sought in the visible region and, finally, if the main characteristic of the application of the glass is to minimize the heat transmitted to the interior, glass with high transmittance in the infrared region should be avoided. Therefore, this analysis of what is necessary in terms of specifying the glazing according to the climate (more or less daylighting; more or less transmission of heat) must be done first and, knowing the transmittance of the glass to be applied in the three regions of the spectrum, it is possible to make the specification more appropriate to the climate.
5. Conclusions
According to the results of the spectrophotometric tests, the double Glass B (green solar control)—Glass E (extremely low-e) (glazing 04) has shown a substantial transmission decrease in all regions of the spectrum, especially in the visible regions (25.2%). On the other hand, the double Glass C (self-cleaning)—Glass G (clear float annealed) (glazing 07) transmits 70.3% of the visible light, which guarantees better conditions of daylight.
The green double Glass B—Glass E (glazing 04) led to the lowest transmission value in the infrared (2.6%) regarding heat. Therefore, such glazing can be efficient in the decrease of the nominal needs of cooling. Double Glass C—Glass G (glazing 07) led to a transmission similar to that of low-e (47.9%) in terms of infrared radiation, which indicates its use will cause stronger heating in an indoor habitable space.
As the climate in Portugal is temperate, the ideal glazing should work as a barrier against ultraviolet radiation and enable the passage of the visible light to favor daylighting. In other words, such glazing should enable good transmission in the visible region and a small amount of heat from the near-infrared so as to help the heating of the indoor environment. Low-emissivity glazings display those characteristics as they usually show good thermal performance for temperate climates. The results of high transmission in the infrared for some materials analyzed show their adequacy for use in countries of predominantly cold weather.
Each type of glazing has led to distinct transmissions for each band of the solar spectrum. The conception of a good daylighting system and thermal comfort requires attention regarding the localization and orientation of the building, but also the variations of the daylight in function of the seasons of the year, time, and weather conditions.
The results of the spectrophotometric tests show defining transmission as adequate only in function of the luminous transmission (in the visible region) of the glazing may not be correct, as the transmission in the other intervals will also influence the thermal comfort. The dimension of the glazed area, its geographic orientation, latitude, and time of the year must also be taken into account. In the evaluation of the daylighting conditions, for instance, the levels of illuminance of an environment can be adequated by the use of glazings of different percentages of visible transmission through the resizing of the windows. The level of illuminance of an environment is related to not only the type of glazing but also to the solar orientation, dimension, and disposition of the opening and the external obstacles, colors of the internal surface, among other factors.
Author Contributions
Conceptualization, H.M.S. and R.C.; Methodology, H.M.S. and R.C.; Formal analysis, L.B. and M.A.; Investigation, H.M.S.; Data curation, H.M.S.; Writing—original draft preparation, review and editing, H.M.S.; Supervision, L.B. and M.A.; Project administration, L.B. and M.A.; Funding acquisition, H.M.S. All authors have read and agreed to the published version of the manuscript.
Funding
This research was funded by Erasmus Mundus External Cooperation Window–ISAC: Improving Skills Across Continents (Framework Partnership Agreement 2008-1021/001 FRAMEECW L16 Coimbra, Specific Grant Agreement 2008-3628/001-001-MUN-ECW).
Institutional Review Board Statement
Not applicable.
Informed Consent Statement
Not applicable.
Data Availability Statement
Data supporting reported results can be found available online in: https://repositorium.sdum.uminho.pt/.
Conflicts of Interest
The authors declare no conflict of interest.