Article Information
- Digital Object Identifier (DOI): 10.47982/cgc.8.469
- This article is part of the Challenging Glass Conference Proceedings, Volume 8, 2022, Belis, Bos & Louter (Eds.)
- Published by Challenging Glass, on behalf of the author(s), at Stichting OpenAccess Platforms
- This article is licensed under a Creative Commons Attribution 4.0 International License (CC BY 4.0)
- Copyright © 2022 with the author(s)
Authors:
- Rena Giesecke - Digital Building Technologies, Institute for Technology in Architecture, ETH Zurich, Switzerland
- Benjamin Dillenburger - Digital Building Technologies, Institute for Technology in Architecture, ETH Zurich, Switzerland
Abstract
The presented research investigates loose sand forming as a reconfigurable molding strategy for custom glass parts. Doubly curved glass parts usually require the labor-intense fabrication of individual ceramic or steel molds. Reconfigurable molds for glass are limited to specific modular geometries and require costly heat-resistant actuation mechanisms. Three-dimensionally (3D) printed sand molds for glass slumping require binders and cannot be reused. The objective of this research is to facilitate a waste-free fabrication of doubly curved glass elements and a facile, fast, low-cost mold-making process for the hot bending of glass. The molding system employs granular loose sand material, which is heat resistant and can be quickly reformed. In combination with novel digital tools and robotic fabrication, the technique provides a flexible molding system for the transformation of industry-ready float glass.
This research presents the first results, including possible granular material systems for loose granular molding, robotic setup and placement strategies for granular materials, and volumetric material formation considering robotic process parameters. Furthermore, it investigates mold stability during slumping and the geometric precision of mold and resulting glass elements. The resulting glass elements are fully transparent with no contamination caused. The presented approach allows for smooth curvatures, easy mold removal, and complete mold recycling without further processing. The method was applied in several mid-scale experiments, including investigations into which family of forms can be produced. The geometric freedom and limitations of the proposed fabrication method are discussed. Reconfigurable sand molding for glass could enable the geometric customization of glass elements and allow for novel optical, structural, or decorative properties in glass facades and windows.
1.Introduction
1.1. Background
Glassmaking has its origins in ancient times. The first evidence of molds for glass goes back to the Roman period. Household objects were made using frit casting, fusing small glass granules under heat inside a mold or casting liquid glass inside a mold (Wight 2011). Before the invention of glass blowing in the first century BC, which enabled the mold-free fabrication of hollow free-form objects (Eisen 1916), artisans used so-called core-forming by wrapping molten glass around a core that would be removed to produce hollow objects (Corning Museum of Glass 2022). Despite the invention of mold-free processes, molds were a highly relevant tool throughout history for the shaping of glass. Mold materials include sand, ceramics, clay-bentonite, graphite, and steel. Among them, sand is a relevant molding material for glass and metal casting.
For the so-called pattern casting process, a wooden pattern is pressed into a sand-clay-bentonite mix and removed before casting (Corning Museum of Glass 2022). While artisans still employ such traditional methods until today for freeform artifacts, the flat glass industry has made significant progress throughout the history of glass engineering for construction. During the Industrial Revolution, the cylinder-blown sheet glass technique enabled the making of flat glass panes for applications in architecture. This process involved blowing a glass cylinder and unfolding onto a flat steel plate to produce flat glass panes (Diderot et al. 2002). The invention of the float glass process in 1952 enabled the production of standardized distortion-free float glass for architecture and the automotive industry (Pilkington 1969). Today, float glass occupies the majority of the glass market, with a continuous predicted annual growth of 4% by 2028 (Statista 2021).
The implementation of curved glass in architecture remains an exception due to the challenge of manufacturing doubly curved glass with standardized quality and high energy and mold costs. Molds for doubly curved glass parts for application in architecture or the automotive industry are usually made of ceramics or chrome steel which are costly and can only be used to produce one type of shape (German Bundesverband Flachglas 2012). Cold bending of glass is gaining popularity due to its ecological advantages. Still, the technique is limited to single and slight double curvatures without the potential to produce small-scale double curvatures within a panel (Fildhuth et al. 2019).
1.2. State of the Art
Recent technologies employ reconfigurable molding systems from chrome steel elements or ceramics to manufacture slumped sheet glass of different shapes with the same mold. However, these are usually limited to specific modular geometries and require costly heat-resistant actuation mechanisms (McGee et al. 2012). The Glass and Transparency Group at TU Delft has presented the first proof of concept for using 3D-printed molds for glass casting as part of a review of different glass molding methods (Oikonomopoulou et al. 2020). Previous research has demonstrated using three-dimensionally printed molds for glass casting, blow molding, and slumping. However, three-dimensional (3D) printed sand molds for glass slumping require binders, cannot be reused, and demonstrate fragility during tests (Giesecke and Dillenburger 2022). Previous research at ETH Zurich has demonstrated the use of a custom tool head for the printing of granular glass (Michopoulou et al. 2021), loose shaping of sand using a robotic setup and a custom dispenser (Medina et al. 2020), and as a reconfigurable molding method for concrete (Gramazio Kohler Research 2022).
1.3. Approach
To enable the implementation of custom doubly curved float glass parts for architecture, this research employs the automated deposition of loose sand as a low-cost, reconfigurable, waste-free molding strategy. The proposed method overcomes the labor-intensiveness and high cost of traditional mold-making techniques, the in-adaptability of 3D-printed molds, and the need for highly engineered reconfigurable molding systems. Reconfigurable molding based on a granular system could enable buildings with glass parts with non-repetitive customized curvature in each part.
2. Method and materials
The following experiments are executed with a CNC setup equipped with a custom tool for sand dispensing. All experiments presented in this paper employ 6mm thick 300x480mm glass panes from the same supplier. The kilning setup is a Nabertherm GF600 glass kiln (Nabertherm 2022). All experiments are carried out with the same heat curve of peak temperatures of 650 degrees Celsius. Materials used for the mold include natural sands and gravel purchased at a construction supplier. More specifically, silica sand at 0.1-0.2mm grain size, sand at 0.35-1.5mm grain size, gravel at 2-5mm size, and rocks at 25-50mm size with no additional materials or binders added, constitute the molding material.
2.1. Granular molding system and kilning process
This chapter investigates the performance of granular molding systems with a range of different materials being tested. Initial tests with cast ceramic parts result in pointy features and the readability of modularity in the glass. Therefore, sand, gravel, and rocks are tested as natural, fully recyclable molding materials that can potentially produce smooth curvatures and be processed with additive manufacturing techniques for the efficient, predictable assembly of the reconfigurable mold.
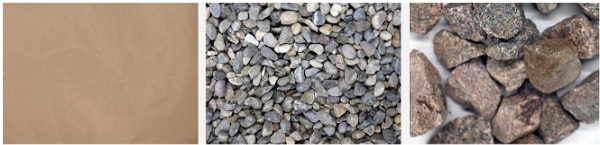
Sand, gravel, and rocks demonstrate great results in heat resistance, recyclability, and removal from the glass part. The resolution and smoothness of the results differ significantly (Fig. 2). Silica sand at 0.1-0.2mm (Fig. 1a) is ideal for shaping geometries with gentle smooth curvature, while gravel at 2-5mm grain size (Fig.1b) and larger rocks at 25-50mm size (Fig. 1c) result in pointy features and are highly dependent on both the unique, rock-specific geometry and its orientation. Due to these findings, the following research focuses on sand as a molding material due to its capacity to shape the glass at high resolution into smooth geometries and the potential of efficiently processing sand using a tool head. However, gravel and rocks show great potential to be placed in locations where sand cannot achieve the desired heights or stability.
In summary, sand is an ideal molding material for the following reasons:
- Heat resistance of approximately 1700°C.
- The sand can be fully recycled (back to its natural state).
- There is no need to add mold release agents or coatings.
- The sand provides a high-resolution system due to its fine granular structure.
- When removed from the kiln, the glass can be easily removed from the sand.
- Sand can be well-processed using a customized tool head.
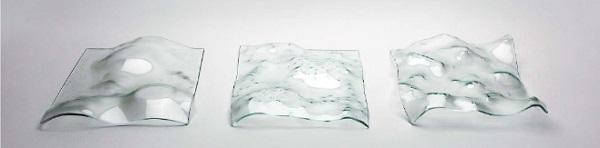
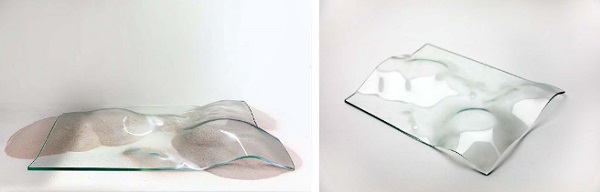
Initial tests with silica sand (Fig. 3) demonstrate that the 6mm flat glass entirely takes the shape of the sand piles and areas where no sand is placed. The placement of the flat glass pane onto the sand piles deforms the tip of the sandpile slightly, causing a flattening of such areas. Sand piles demonstrate excellent stability, assumingly caused by the load of the glass being applied and the jamming of granular material below. The precision of the resulting parts with being further investigated in 2.5 Precision: mold deformation and 3D scanning analysis. For the glass slumping process, the glass pane is carefully placed on top of the deposited sand in the kiln. To form the glass, the kiln is heated up to 650°C and then cooled along several stages to release stresses. Higher temperatures of up to 800°C can potentially be applied for more extreme curvatures or thicker glass panes.
2.2. CNC setup and sand dispenser tool head
The robotic setup for dispensing the sand consists of a CNC motion system or UR10 robotic arm and a customized end effector (Fig. 4). The end effector is designed as a test setup for printing different types and colors of granular materials. The tool head can be attached to a CNC setup or robotic arm. A cylindric pipe provides storage for the sand, which can be refilled from above. The rotating screw consisting of an off-the-shelf drill bit releases the sand and transports it through a 6mm pipe to the tip of the tool head, which consists of a 3D-printed conical plastic part. The volume of sand released can be controlled through motor revolutions. The current setup is calibrated for the sand of 0.35-1.5mm grain size but could be adjusted to different grain sizes by adjusting the screw and pipe diameter.
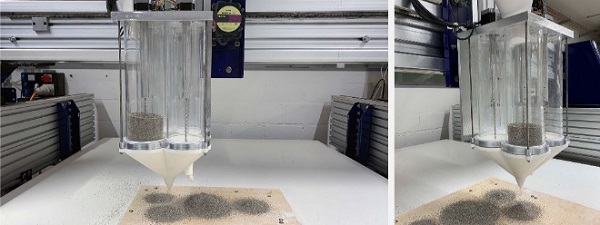
2.3. Process parameter calibration and sand printing
The robotic setup for dispensing the sand consists of a CNC motion system or UR10 robotic arm and a customized end effector (Fig. 4). The end effector is designed as a test setup for printing different types and colors of granular materials. The tool head can be attached to a CNC setup or robotic arm. A cylindric pipe provides storage for the sand, which can be refilled from above. The rotating screw consisting of an off-the-shelf drill bit releases the sand and transports it through a 6mm pipe to the tip of the tool head, which consists of a 3D-printed conical plastic part. The volume of sand released can be controlled through motor revolutions. The current setup is calibrated for the sand of 0.35-1.5mm grain size but could be adjusted to different grain sizes by adjusting the screw and pipe diameter.
Table 1: Process parameters.
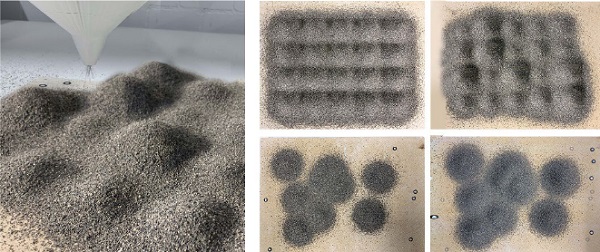
2.4. Precision: Mold deformation and 3D scanning analysis
This chapter investigates the precision of resulting glass samples in relation to the scans of the loose sand mold. To identify the deformations resulting from the glass pane being placed onto the loose sand and the slumping process in the kiln, the sand is scanned before and after the kilning process. The sand is 3D scanned using a GOM ATOS CORE 300 scanner with a precision of 10-20μm and the data comparison is conducted with GOM Inspect software. Fig. 6 shows minimum deviations from the target geometry resulting from the weight applied when placing the glass pane onto the loose sand. While the overall sand geometry results in high precision, the tips of sandpiles show deformations of up to 2mm (Fig. 6, visualized in red) resulting from the load applied when placing the glass. Through this process, the sand is pushed downwards, resulting in deviations of less than 2mm below the tip of the sand piles (Fig. 6, visualized in blue).
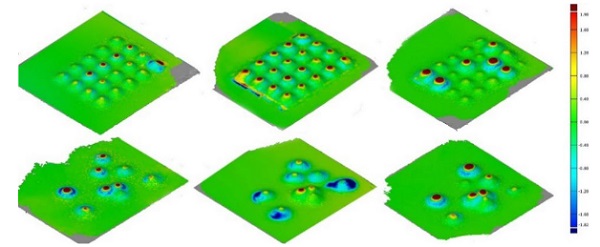

2.5. Glass slumping tests
Fig. 7 shows that the glass pane entirely shapes onto the loose sand typology, including the flat areas where no sand has been placed. The glass demonstrates optically transparent properties without contamination from the sand or process. The granular material is imprinted into the glass depending on the sand size. Fig. 7b shows the resulting patterns in the glass with punctual deformations along a regular grid of constant height that demonstrates the repeatability of the presented process, varying heights, an irregular grid of constant heights, and an irregular grid of varying heights with the resulting reflective and refractive properties of the deformations in the glass (Fig.8).
2.6. Families of forms: Geometric freedom and limitations
The families of forms that can be produced with the presented process are highly dependent on the jamming behavior of the sand and the automation method applied. The current approach of using fine sand without any additional material or binder is suitable for smoothly transitioning geometries and soft curvatures in doubly curved surfaces. Limits in heights, scale, and curvature extremities will have to be investigated in further tests. Combining rocks and sand might help produce geometries with more extreme curvatures and stabilize the loose sand typology through strategic placement.
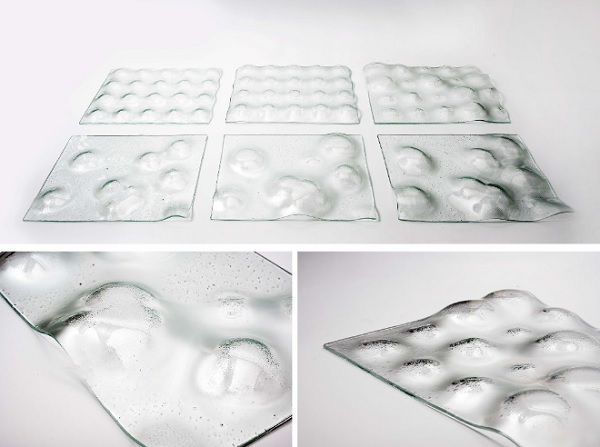
3.Contributions
The novel, waste-free molding system: This research extends the state of the art by providing a novel, waste-free, reconfigurable molding system for doubly curved glass. Mid-scale experiments provide the first proof of concept for combining the automated shaping of loose sand to manufacture doubly curved glass parts. The experiments above have demonstrated the potential of using loose sand to shape glass and printing with sand for this purpose in an automated manner.
Novel robotic dispenser for granular material: The novel robotic dispenser enables the controlled automated distribution of granular material. It provides a custom tool head solution compatible with CNC or robotic arm motion systems and enables the precise assembly and reconfiguration of loose material.
Reconfigurability and recycling: While molds for glass usually require casting or milling processes of one final shape or modules and complicated automated reconfiguration mechanisms, the loose molding material can be fully recycled and reshaped; it provides a glue and waste-free molding technique for glass based on a natural material.
Resolution and precision: Sand provides a high-resolution material system for molding with high precision of only 2mm deviations in local points from the target geometry. Simulation and volumetric modeling could further mitigate these deviations and predict them within the design-to-production workflow.
Cost and accessibility: With access to a CNC setup or robotic arm and glass kiln, this technique is easily accessible at a low cost. The molding material comes at almost no cost, and the prototypical custom tool head (one channel is sufficient) can be built for approximately 300 Euros for electrical components, 3D-printed parts, and acrylic pipes.
4.Discussion
Digital freeform glass: In architecture, glass is usually implemented as a standardized, flat material. The glass industry has mostly neglected shape customization due to the high costs of producing freeform glass and the requirements for expensive molds. AM can contribute to the predictable design and fabrication of digitally crafted glass, potentially enabling economically viable glass customization.
The novel, waste-free molding system: Accessibility and novel design space for glass. Loose sand molding provides a low-cost, easily replicable method for shaping glass three-dimensionally without the requirement for producing molds from ceramics or chrome steel that are hard to handle, break easily, and require labor-intensive casting processes. This research opens up a new design space for designers, architects, and engineers to produce new properties in glass parts. Potential features include reflective, refractive, and structural properties in the glass.
Reconfigurable granular material system and process-aware design: The reconfigurability of the material system, material interactions, and process implemented substantially impact the families of forms produced. It is highly relevant to produce process and material-aware design approaches. Computational design and additive manufacturing are highly efficient tools for designing with such systems.
Computational volumetric modeling: To improve the predictability of the presented AM method, computational design and volumetric modeling, such as volumetric modeling for terrain implemented in landscape modeling (Hurkxkens 2019), could support a stable design-to-production workflow that considers the physical parameters of the process and limitations of the material system.
Upscaling and applications: The presented research provides a small-scale proof of concept. It is crucial to address challenges relevant to upscaling and construction, including the feasibility of upscaling the fabrication process to large glass panes, the joinery of freeform parts into architectural facades, and the structural performance of parts. Applications could include windows for façade panels that redirect light for functional or decorative purposes or have specific structural properties resulting from curvature.
Limitations and challenges: Challenges in the production process include the quality control of kilned glass parts. It remains an open question how the material structure is affected by the kilning process and how the doubly curved float glass behaves structurally compared to off-the-shelf float glass.
Future work: Future work will include lab testing to determine the performance of the resulting parts in terms of structural properties and investigate the joinery of unique doubly curved glass parts. Furthermore, future work will explore how functional structural and optical properties can be produced to demonstrate new properties in glass architectures and facades.
Acknowledgements
The authors acknowledge the technical support of Tobias Hartmann from the Robotic Fabrication Lab (RFL) and Robert Presl from the Institute of Geodesy and Photogrammetry at ETH Zurich. On behalf of all authors, the corresponding author states that there is no conflict of interest.
References
Corning Museum of Glass: Core Forming. Corning Museum of Glass Dictionary. https://www.cmog.org/glass-dictionary/core-forming. Accessed 18 June 2022
Corning Museum of Glass: Sand Casting. Corning Museum of Glass Dictionary. https://www.cmog.org/glass-dictionary/sand-casting#:~:text=A%20forming%20technique%20in%20which,into%20contact %20with%20the%20sand. Accessed 18 June 2022
Diderot et al.: L`Encyclopedie - Il Vetro. Libroitalia, Italy (2002)
Eisen, G.: The Origin of Glass Blowing. American Journal of Archaeology 20(2), 134–143 (1916)
Fildhuth, T., Oppe, M., Schieber, R.: Entwerfen und Konstruieren mit gekrümmtem Glas. In: Weller, B., Tasche, S. (eds.) Glasbau 2019 - Bauten und Projekte. Bemessung und Konstruktion. Forschung und Entwicklung. Bauprodukte und Bauarten, pp. 59-74. Wiley-VCH, Dresden (2019)
German Bundesverband Flachglas: Guidelines for Thermally Bent Glass in the Building Industry. BF Bulletin 9 (2012)
Giesecke, R., Dillenburger, B.: Three-dimensionally (3D) Printed Molds for Custom Glass Parts. In: Belis, J., Louter, C., Nielsen, J.H., Schneider, J., Overend, M. (eds.) Glass Structures & Engineering (2022). https://doi.org/10.1007/s40940-022-00176-y
Gramazio Kohler Research: Procedural Landscapes 2. https://gramaziokohler.arch.ethz.ch/web/e/lehre/211.html (2011). Accessed 10 Janurary 2022
Hurkxkens, I., Bernhard, M.: Computational Terrain Modeling with Distance Functions for Large Scale Landscape Design. In: Buhmann, E., Ervin, S., Helh-Lange, S., Palmer, J. (eds.) Journal of Digital Landscape Architecture no. 4. Wichmann Verlag, Berlin (2019)
McGee, W., Newell, C., Willette, A.: Glass Cast - A Reconfigurable Tooling System for Free-Form Glass Manufacturing. In: Acadia Conference Proceedings, pp. 287-294 (2012)
Medina Ibáñez, J., Jenny, D., Gramazio, F., Kohler, M.: Feedback Formation: Three teaching projects on robotically manipulated granular materials. In: VIII Jornadas sobre Innovación Docente en Arquitectura, pp. 59-69 (2012)
Michopoulou, S., Giesecke, R., van den Bulcke, J., Odaglia, P., Dillenburger, B.: Robotic Grading of Glass – Additive Manufacturing of Heterogenous Color and Transparency. In: Globa, A., van Ameijde, J., Fingrut, A., Kim, N., Lo, T.T.S. Reflections- Proceedings of the 26th CAADRIA Conference – Volume 1 (2021), pp. 563-572 (2021)
Nabertherm: Fusing Furnaces. https://nabertherm.com/en/products/arts-crafts/fusing-furnaces. Accessed 8 Feburary 2022
Oikonomopoulou, F., Bhatia, I.S., Damen, W., Van Der Weijst, W., Bristogianni, T.: Rethinking the Cast Glass Mould - An Exploration on Novel Techniques for Generating Complex and Customized Geometries. In: Belis, J., Bos, F., Louter, C. (eds.) Challenging Glass Conference Proceedings vol. 7, pp. 155–174. TU Delft Open, Delft (2012)
Pilkington, L.: Review lecture: the float glass process. In: Proceedings of the Royal Society of London. Mathematical and Physical Sciences 314, pp. 1–25 (1969)
Statista: Glass industry. https://www.statista.com/study/43866/glass-industry/. Accessed 12 December 2021
Wight, K.B.: Molten Color: Glassmaking in Antiquity. Springer, Los Angeles (2011)