Authors: M Čeněk and D Hlaváček
Source: IOP Conference Series: Earth and Environmental Science, Volume 290, Central Europe towards Sustainable Building (CESB19) 2–4 July 2019, Prague, Czech Republic
DOI: 10.1088/1755-1315/290/1/012114
Abstract
Built environment is one of the major causes of the negative impact that human activity has on the environment. Building design should adopt such strategies that would help relieve this situation. Architects should therefore be able to grasp and implement all aspects of sustainable design, including cutting edge technologies, in a holistic and integral fashion. Building-Integrated Photovoltaics (BIPV) represents an important field to explore, since photovoltaic systems have an enormous potential within the context of architectural and urban design. Their implementation though has to be part of the integral design process which is essential for the creation of quality sustainable architecture. Since the world urban population is constantly growing, sustainable approaches to architectural design need to be adopted with urgency in urban environment.
Existing urban environment however presents great challenges to the implementation of any new technology. In an urban context we need not only design new buildings, but since the urban tissue is already in place, care must be taken not to damage the historical and architectural values. Furthermore, urban environment has many variations and offers a broad scope of possibilities for various approaches to BIPV. The aim of this paper is to demonstrate the possibilities of BIPV in relationship to sustainable architecture and urban environment, focusing on explaining the necessity to provide architects with a methodology of working with advanced photovoltaic systems in architectural design, introducing the departure points for this intention, and demonstrating how such a methodology – a Design Manual – will be created.
1. Sustainability and Architecture
1.1. Environment and building industry
Already in the 1960´s American architect and visionary Richard Buckminster Fuller stated that “the Earth is a mechanical object just like an automobile, therefore we need to refill oil, fuel, water for radiator, and take care of it as a whole” [1].
The rising number of inhabitants on the planet and their striving for improved living standards is creating enormous pressure on the environment. The built environment participates significantly in this situation. In the developed world the building industry accounts for 40% of the total energy consumption [2] and around 40% of all human-produced waste. At the same time, it is responsible for approximately 30% of CO2 emissions [3]. It is obvious that to reduce the impact of human activities on the environment a change in approach to building design is needed in order to adopt sustainable design strategies.
As architects we take on the responsibility for the built environment and its quality. Architects should be able to grasp and implement all aspects of sustainable design in a holistic and integral fashion.
1.2. Sustainable architecture and integral design
Sustainable architecture is a fundamental topic, a consequence of decisions made during the design process. Since architects need to be able to address and coordinate all aspects of a design, they should evidently be aware of the existing, as well as arising, technical and technological possibilities available to them. „Sustainability is an opportunity to ask old questions in a new way.” [4]
Ignoring technological progress is not the correct way to approach sustainable design, although in some cases it may look very tempting, and a considerable percentage of architects as well as the general public still today tend to interpret sustainable (or “green”) architecture as a substantially lowtech issue [5]. At the same time however, sustainable architecture should not be dependent exclusively on technologies, nor should these be a mere “add-on”.
For architects however, technologies are often a design feature which proves difficult to tackle and implement successfully. Technologies thus frequently remain a separate field which reduces the quality of the building but often also the quality and efficiency of the technologies themselves. This limitation creates the need for an interdisciplinary team to take part in the design process from the very beginning – from the conceptual design phase.
In sustainable architectural design, technologies should always represent a “natural” and ingenuous part of the overall process and result. Integral design seems to be the only correct path to sustainable architecture and sustainable built environment.
2. Building-integrated Photovoltaics
2.1. Energy and landscape
“Civilization requires energy, but energy use must not destroy civilization!” [6] Energy is one of the key issues of the environmental (ecological) pillar of sustainable development (with the other two being economic and social). The way mankind manages energy and what sources are used to produce this energy has direct consequences on the ecology of the Planet, but equally it affects the world´s economy and society. When thinking about energy, it is essential to have in mind that approximately 1 billion of the world´s population still does not have any access to electrical energy. [7]
Furthermore, the European Union´s climate package for 2020 works with a “20-20-20” strategy on climate and energy meaning that as a whole, the EU has set three key targets:
- „20% cut in greenhouse gas emissions (from 1990 levels)
- 20% of EU energy from renewables
- 20% improvement in energy efficiency“ [8]
For 2050 the objectives are even more ambitious – to reduce greenhouse gas emissions by 80–95% compared to 1990 level [9]. If we were to meet these goals by producing all electrical energy from solar radiation only this would require the installation of about 5,000 square kilometres of photovoltaic panels over the following 40 years, i.e. a surface equalling to about 0.1% of the area of the EU.
Shrinking of natural landscape due to man-made construction is yet another threat to the environment we live in. It has increased rapidly over the last decades, mainly due to massive and unceasing urbanisation. In EU only, man-made surfaces (mostly built-up areas and infrastructure) make over 4% of total land surface, 180,000 square kilometres in total [10]. Large scale groundmounted PV installations unfortunately play an increasingly important role in this process as well. Not only do they contribute to the reduction of the production areas for agriculture, but also to the loss of open countryside along with its cultural, ecological and aesthetic functions. At the same time, they produce electrical energy far from the main places of consumption – concentrated urban areas.
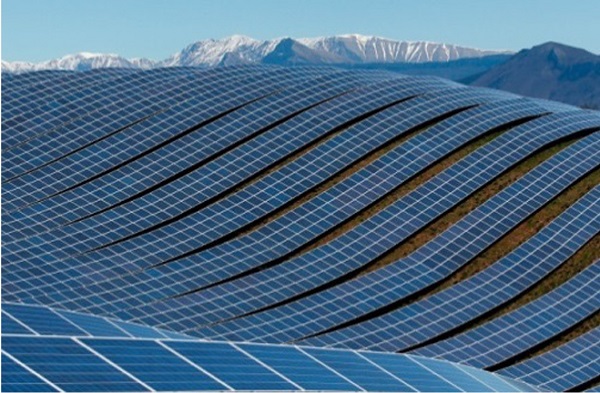
2.2. Urbanism and Photovoltaics
Constant urbanization generates the need for the creation of truly sustainable cities. “Today, 54 per cent of the world’s population lives in urban areas, a proportion that is expected to increase to 66 per cent by 2050.” (…) The urban population of the world has grown rapidly from 746 million in 1950 to 3.9 billion in 2014. (…) The world’s urban population is expected to surpass six billion by 2045“ [11].
These alarming figures prove that the built environment and its needs will in the near future continue to play a core role with respect to sustainable development. “Managing urban areas has become one of the most important development challenges of the 21st century. Our success or failure in building sustainable cities will be a major factor in the success of the post-2015 UN development agenda” says John Wilmoth, Director of UN DESA’s Population Division [12].
Photovoltaic systems are today a mature technology that has an enormous potential within the context of the cities: on roofs, façades, transparent and semi-transparent elements and constructions, complementary structures or transport and technical infrastructure. This is especially evident for “Building-integrated Photovoltaics” (BIPV) which can be fully aesthetically and structurally integrated in buildings and other structures and thus become an integral part of the urban environment in order to provide as much energy as possible. Photovoltaics in general represents one of the technologies that can in the near future have a strong impact on the image of the buildings and the cities we live in.
2.3. Building-integrated Photovoltaics
“More than just providing electrical energy, BIPV can enhance and satisfy a building image. BIPV makes a statement about innovative architectural as well as engineering design” [13]. Implementing photovoltaics in urban environment however has its challenges which originate from the dense and diverse city fabric (orientation, form, massing, layout, height of surrounding buildings, total space available), social (acceptance of new material with specific aesthetic quality) and cultural aspects (use of photovoltaic systems in city conservation areas, i.e. zones of special architectural or historic interest, the character and appearance of which should be protected and preserved) of the urban environment.
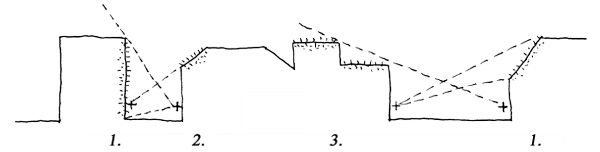
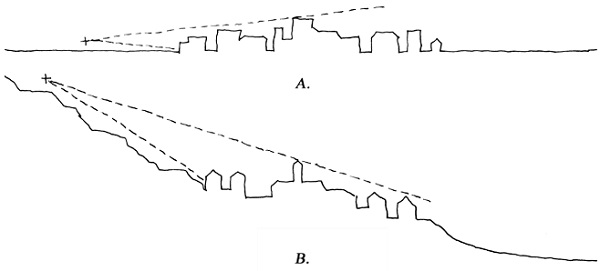
Not only technical requirements of photovoltaics, such as the influence of orientation, albedo and overshadowing, ventilation possibilities, dirt accumulation etc., but also its characteristic aspects (dependent of the particular technology used) such as colour effects, transparency, reflection or flexibility have to be taken into account. And, most importantly, as Maria Cristina Munari Probst and Christian Roecker argue [14], architectural quality should not be sacrificed “to promote solar spread”. In their work they come forward with the notions of “architectural integration quality”, which is essential in urban environment, and “architectural criticity” which is based on visibility criteria – close and remote visibility of city surfaces, as illustrated in figures 2 and 3 and sensitivity of urban context.
With more sensitive contexts and high visibility of the photovoltaic (and other solar) systems, higher quality of design integration is needed.
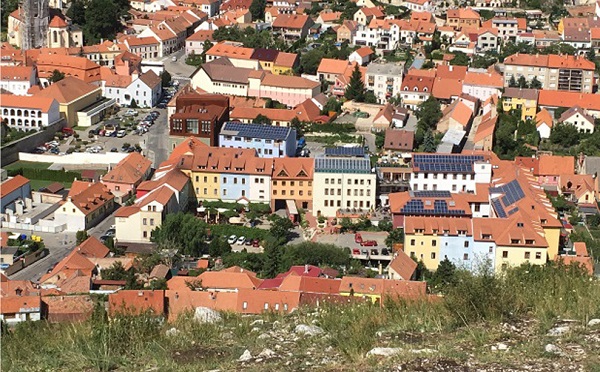
When designing with BIPV architects have a number of opportunities how to integrate the currently existing photovoltaic solutions into the building design. The main possibilities include shading systems, rain screen (cladding) systems, curtain walls, double skin façades, application on atria and canopies, as well as progressive glazing solutions. Advanced photovoltaics can be integral part of more traditional building elements, such as roof tiles. The basic levels of integration can range from merely added technical elements (suitable mainly for low visibility and low sensitivity contexts) to fully optimized architectural form (suitable for specific new build cases) as illustrated in the scheme in figure 5 [15].

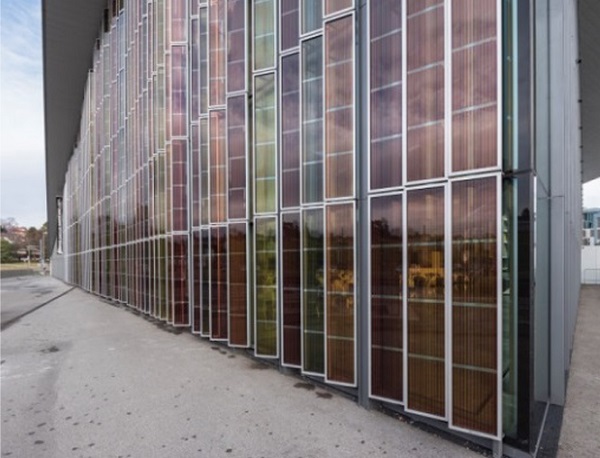
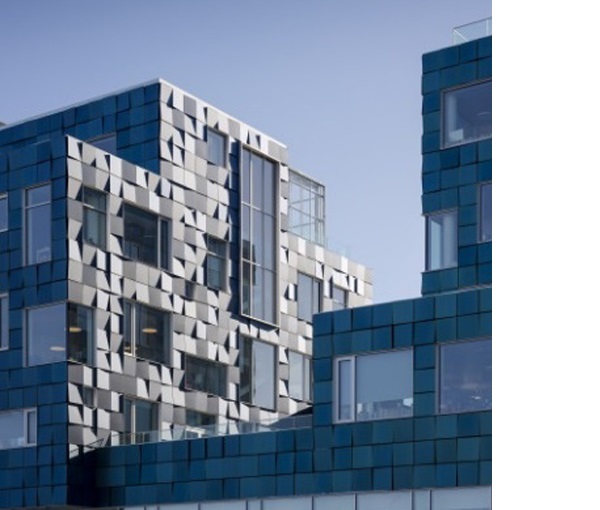
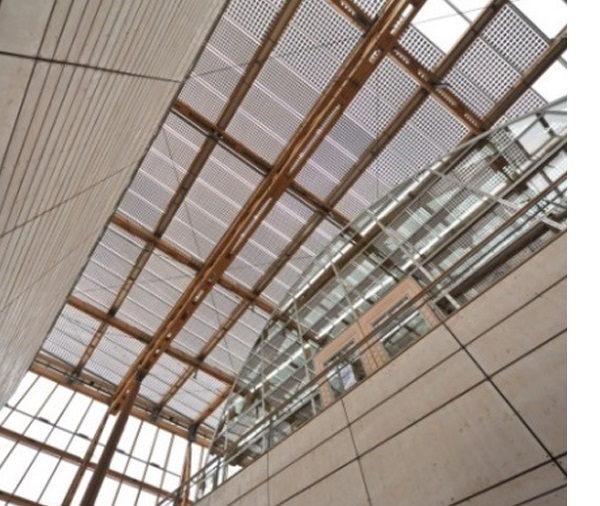
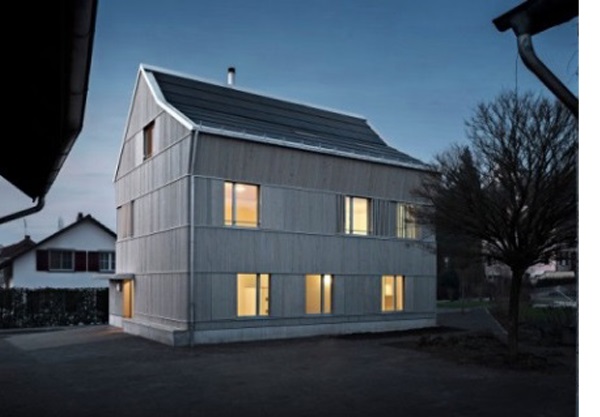
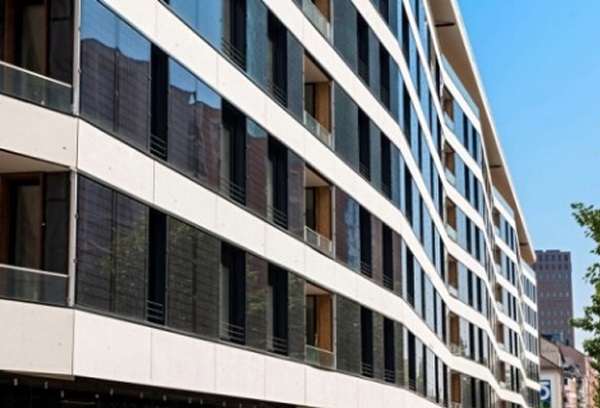
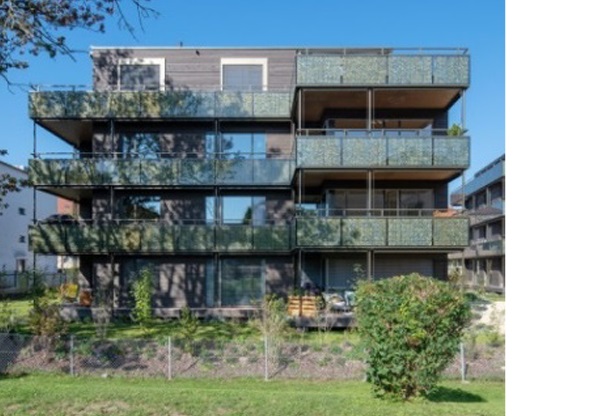
To sum up, BIPV can represent a possible solution to rising energy needs, land depletion and constant urbanization, at the same time becoming an architectural element of sustainable architectural design.
3. BIPV Design Manual
3.1. Design Manual
The goal of the architects from the Department of Architectural Design II of the Faculty of Architecture engaged in the project of the Centre for Advanced Photovoltaics and working on the topic of BIPV is to create a “Design Manual” that would aid the design process and address some of the most demanding architectural issues in successfully applying – and integrating – photovoltaics in architecture in a simple and clear way. The Design Manual is to be completed and published by the end of 2020.
3.2. Data
Collaboration with the specialists from the Faculty of Civil Engineering of the Czech Technical University in Prague, as well as experts on micro grids from University Centre for Energy Efficient Buildings, provides the necessary technical data based on detailed studies on building physics and grid phenomena. The energy needs, usage patterns, demands etc. of existing buildings in real urban situations are being analysed (for an example of such data see section 3.3). This data helps to identify suitable surfaces for PV application and possible and optimum energy coverage and hence allows architects to draw conclusions and suggest specific approaches to BIPV design in typical simplified situations and estimate, depending on the technology used, to assess their economic feasibility. The methodology of data collection and respective preliminary results are not part of this paper, they are presented separately by their authors.
3.3. Model urban locations
Urban environment can have many variations and offer a broad scope of possibilities (and limitations) for various approaches to BIPV. Also, in an urban context, we need not only design new buildings, but right the opposite – the urban texture is already in place. In a sustainable city, existing buildings need to be re-used, their existence protected and their form updated with the utmost care, in such a way that their value and the value of the urban context are not damaged.
Prague was chosen for the work for its richness of typologies and urban fabric, ranging from heritage UNESCO protected historical centre, to various present-day suburban situations, and its needs to develop and face the challenges of the future. Prague can also serve as a representative of many Central European cities.
Using available data from the Institute of Planning and Development of the City of Prague typical contexts within the city of Prague were identified. These model locations and appropriate building typologies and forms represent typical scales and densities of urban tissue:
- Historical (medieval) city (e.g. parts of Staré Město) (dense and irregular structure, uneven orientation, small surfaces, complex usage, high historical value, very high visibility, high energy demand etc.)
- Compact city (19th century block tissue, e.g. Vinohrady) (regular but still dense structure, predictable orientation, larger surfaces, complex usage, high historical value, high visibility, high energy demand etc.)
- Modernist city (1960–90's, e.g. Jižní Město) (very regular and sparse structure, regular orientation, large scale surfaces, easily predictable usage patterns, energy needs possible to be reduced by other technical means etc.)
- Garden city within urban core (e.g. Ořechovka) (sparse low rise structure with relatively even orientation, small surfaces, relatively predictable usage and relatively low energy demand, high historical value and visibility etc.)
- Low rise suburb (example of urban sprawl) (sparse low rise structure with low overall quality and energy needs possible to be reduced by other technical means)
- Commercial suburb (very large regular low rise structure with huge horizontal surfaces, predictable usage, high demand)
The historical centre of Prague is an example of a situation where both visibility and sensitivity are the highest, at the same time the dense and organic urban fabric presents a complicated situation from the technical point of view. Very careful and detailed work, such as the update of small-scale building elements (such as roof tiles) seems to be the most plausible way of BIPV application. Future possibly lies in new materials, such as photosensitive mortar additives in order to create energy generating rendered surfaces, or fully transparent PV. New build structures in historical centre have to respect their context but offer a greater range of possibilities including facade cladding or PV roof cladding, shading elements etc. (as illustrated in figures 7 and 9).
Modernist city, by contrast, seems to be an ideal background for extensive BIPV usage and experimentation – both due to the planning regulations which were at the basis of the layout of such districts (buildings far from one another to avoid overshadowing, orientation almost exclusively southnorth or east-west), and the relatively simple (in terms of architectural language) form of the buildings. Apart from obvious (non-BIPV) applications on flat roofs, PV balconies, PV shading elements, but also entire PV facades can be envisioned in such a context (such as in figures 10 and 11).
Commercial suburb, clearly at the other end of the spectrum, is a low sensitivity example, where simple, yet extremely effective and efficient PV installations are possible.
Based on the data currently available (compact city – Vinohrady and modernist city – Jižní Město), it is obvious that implementation of photovoltaics from energy point of view is only really useful on a relatively small portion of the actual buildings´ surfaces: effective surface percentages for buildings vary between 5–18% in Vinohrady and 15–27% in Jižní Město respectively. This knowledge has to be taken into account when considering the various ways in which BIPV can be used in the model situations [16].
4. Conclusion
By generalizing the lessons learned from the described preparative and analytical work, a methodology of design and assessment of BIPV in the early stage of an architectural concept in urban context is going to be created. It is particularly important to provide architects a clear Design Manual applicable from the very start of the design process, because the conceptual phase of the design has major impact on all the following design phases and thus on the quality of the resulting building as well as its surroundings, and in consequence the whole environment.
The Design Manual, apart from introducing to architects the available technologies, theis limits, challenges and drawbacks and providing the necessary theoretical background on BIPV will provide specific case study solutions (recommended design approaches) illustrated by examples of good practice based on the three main aspects described, i.e.:
- The architectural and historical quality of the urban tissue, its visibility, architectural and social limits, based on an architectural analysis of the context;
- The energy demand and energy production possibilities of the particular context and technical limits, based on modelled and calculated data and analysis of building volumes and usage;
- Available BIPV technologies.
Acknowledgments This article and the work described has been supported by the Operational Programme Research, Development and Education of the European Structural and Investment Funds, project CZ.02.1.01/0.0/0.0/15_003/ 0000464 Centre for Advanced Photovoltaics.
References
[1] Fuller R B 2008 Operating Manual for Spaceship Earth (Baden: Lars Müller Publishers)
[2] U.S. Department of Energy 2012 Buildings Energy Data Book (online) available at http://buildingsdatabook.eren.doe.gov/ChapterIntro1.aspx
[3] CIB 1999 Agenda 21 for Sustainable Construction (CIB Report Publication)
[4] Villemard J 2013 Headquarters of the CFE/Rubelles what makes it sustainable Interior Design and Eco Architecture (Prague: IDEA)
[5] Cílek V 2008 Stavět podle přírody nebo podle člověka? Zelená architektura.cz (Prague: GJF a Architektura) p 31
[6] Pope Francis 2018 Address of His Holiness Pope Francis to participants at the meeting for Executives of the main companies in the oil and natural gas sectors, and other energy related businesses (online) available at: http://w2.vatican.va/content/francesco/en/speeches/2018/june/documents/ papafrancesco_20180609_imprenditori-energia.html
[7] International Energy Agency 2017 Energy Access Outlook 2017 (online) available at: http://www.iea.org/access2017/
[8] European Commission 2018 2020 Climate & Energy Package (online) available at: https://ec.europa.eu/clima/policies/strategies/2020_en
[9] European Commission 2018 2050 Energy Strategy (online) available at: https://ec.europa.eu/clima/policies/strategies/2050_en
[10] Eurostat 2018 How much of your region is covered by man-made structure? (online) available at: https://ec.europa.eu/eurostat/web/products-eurostat-news/-/WDN-20180523-1 [11] UN 2014 World’s Population Increasingly Urban with more than Half Living in Urban Areas (online) available at: http://www.un.org/en/development/desa/news/population/world-urbanization-prospects2014.html
[12] UN 2014 World’s Population Increasingly Urban with more than Half Living in Urban Areas (online) available at: http://www.un.org/en/development/desa/news/population/world-urbanization-prospects2014.html
[13] Roberts S and Guariento N 2009 Building Integrated Photovoltaics: A Handbook (Munich: Birkhauser Verlag) p 11
[14] Munari-Probst M C and Roecker C 2012 SolarEnergy Promotion & Urban Context Protection: LESO-QSC (Quality-Site-Visibility) Method (Bologna: PLEA 2015)
[15] Farkas K, Frontini F, Maturi L, Roecker C, Scognamigliio A and Zanetti I 2012 Photovoltaic Technologies Solar Energy Systems in Architecture – integration criteria and guidelines eds Munari-Probst M C and Roecker C (Report T.41.A.2: IEA SHC Task 41 Solar energy and Architecture) p 96
[16] Skandalos N, Tywoniak J, Maierova L and Stanek K 2018 Assessment of the BIPV potential at the city of Prague and their effect on the built environment (Syracuse: IBPC2018)