AUTHORS:
Helen E Sanders, Vice President, Technical Business Development, SAGE Electrochromics, Inc., Faribault, MN,
Louis Podbelski, Vice President, Architectural Solutions, SAGE Electrochromics, Inc., Faribault, MN
1. ABSTRACT
High performance sustainable building standards such as ASHRAE 189.1, IgCC and LEED recognize the need for windows to provide natural daylight and views, yet this requirement is often at odds with the need to reduce building energy and can impact occupant comfort creating a significant design challenge.
Indeed there is significant controversy currently over data that suggests that buildings meeting these sustainable building standards do not actually have lower energy usage than conventionally designed buildings. This is adding additional fuel to arguments for the reduction of allowable window area in both baseline and green building codes.
Electronically tintable glass can offer a solution which avoids having to trade off daylight and views with energy performance and occupant comfort, allowing more glass to be used without energy penalty and without causing thermal or visual discomfort for occupants.
This paper reviews a range of new case studies where dynamic glazing has been used to solve acute solar control problems through retrofits and used in new high performance designs where the architect has been able to execute on their design vision of a highly glazed building without having to compromise either energy performance or occupant comfort. In addition it reviews some key advancements in EC glass design and performance which is providing enhanced architectural design freedom.
2. INTRODUCTION
From the very beginning of architecture, man has sought to bring sunlight into his buildings and thus was born the window. For centuries, buildings have been designed in clever ways to maximize the admission of daylight, for that was the only source of light. However, in the last century or so, with the advent of electric light, building design has changed to create deeper floor plates and interiors solely lit with electric light.
The resulting “cube farm” with office workers toiling for hours at a time in windowless areas has been an all too common feature of our working environment. As a result, in the last century access to daylight has declined in our built environment.
The more we understand about the impact of changing daylight patterns on the body’s circadian rhythms and the influence of daylight and views on the health, well-being and productivity, the more we understand about the importance of daylight in buildings.
There is an increasing body of evidence that is demonstrating significant health risks related to the disruption of the circadian rhythms because of lack of daylight exposure at the right time of the day or too much of the wrong color light at the wrong times (see for example Blau 2014, Schernhammer et al. 2001, White et. al 2013, Ancoli-Israel et al. 2012). Such risks include:
- Increased risk of obesity
- Increased risk of cancer
- Reduced immune system strength
- Increased risk of heart disease and diabetes
- Increased risk of depression/aggression
A recent study has shown that night shift health care workers suffer from higher rates of obesity, lower fertility, and higher risk of cancer than their day shift counterparts (Schernhammer et al. 2001; Schernhammer 2014).
A recent article in the Economist (Blau, 2014) suggested that the epidemic in attention deficit disorder in children could be a result of lack of sleep, caused by circadian rhythm disruption from not enough daylight in the morning and too much screen time (blue light) at night.
Since people are now spending 90% of their day inside buildings the quality of the built environment is becoming more and more critical to human health.
Green building codes and standards such as BREEAM in the UK, the U.S. Green Building Council’s LEED program, which is codified through IES/ANSI/ASHRAE 189.1, and the International Green Construction Code (IgCC), recognize this issue and that a sustainable design is not just about energy efficiency.
All of these standards have sections that require the provision of daylight and views to the outside. However, the provision of more daylight can come with unintended consequences to occupants in the form of thermal and visual discomfort. This is often due to too much heat gain and glare coming through the windows since the conventional static building envelope can’t respond to the ever-changing exterior environment.
As such, the benefits of access to natural daylight can often be offset by thermally and visually uncomfortable spaces. In the case of glare, occupants pull blinds and shades and then block the admission of daylight and the view to the outside, negating the benefits of daylighting and views.
The use of manual blinds also causes the building energy use to go up compared to the design loads because of the increased use of electrical lighting to compensate for the fact that the blinds remain drawn long after the glare condition has gone.
Indeed, in recent years, it has come to light that a number of green certified buildings are actually not any more energy efficient than their non-green designed counterparts. This has led to the initiation of energy performance verification requirements of the as-built designs.
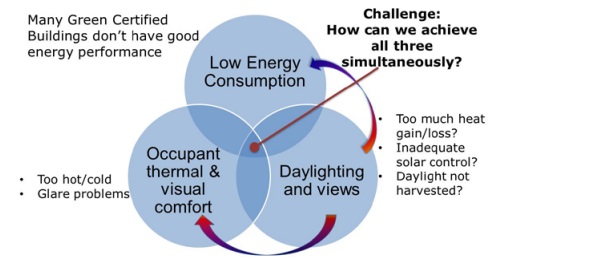
In parallel, the push for increased energy performance in buildings has also given rise to significant debate about the role of windows, and more importantly, the amount of glass that should be used (Wilson 2010; Shuttleworth 2008). Windows are often seen as the weak link in a building because of their lower insulation performance and higher solar heat gain compared to a solid wall.
Indeed, in both Europe and North America we are seeing an increasing trend to reduced window area in new building codes through increasing stringency of insulation values and whole building energy efficiency targets (Part L of the Building Regulations in the UK), or through specific window area limits as in the International Energy Conservation Code (IECC) 2012.
These trends are also being mirrored in the green codes as demonstrated very recently by the highly public debate over the proposed reduction in the allowable window area from 40% to 30% in the prescriptive path of ASHRAE 189.1 (Devlin 2014; Sanders 2014). After significant pushback from the daylight design and research community as well as the glass industry it was withdrawn, but the debate continues.
The window to wall ratio debate within the green codes especially is leading to a dichotomy between providing acceptable window area for daylighting and views and achieving energy efficiency targets, all while providing occupant visual and thermal comfort. This is a major challenge for “green building” design as is pictorially represented in Figure 1.
3. AN ELEGANT SOLUTION
Electrochromic (EC) glass, which can, at the touch of a button or command from a building automation system, modulate its solar heat gain coefficient (SHGC) and visible light transmission over a wide range, stopping at points in between (e.g. a range of 60% to 1% in visible light transmission and 0.41 to 0.09 in SHGC), can provide an elegant solution to this problem (see figure 2).
EC glass saves energy in all climate zones by providing passive solar gains during heating seasons, minimizing cooling loads during cooling seasons and providing maximum daylight harvesting potential, replacing the use of electric lights with natural light in all seasons.
Additionally, because products today can achieve transmissions of 1%T or less, they can control glare without using blinds, thus preserving the view and connection with the outside in contrast to mechanical alternatives which block or mar the view. Studies have shown that 1%T is required to be able to control the glare (LBNL 2006; Kelly et al. 2013).
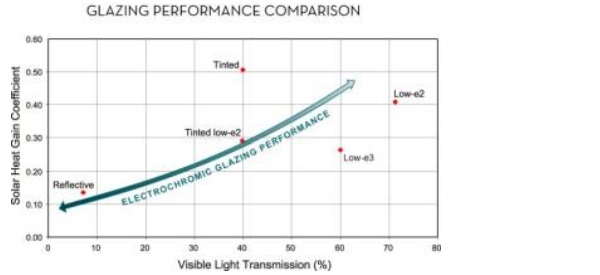
EC coatings can be cleanly integrated into a double or triple glazing unit just like traditional coatings (see Figure 3) and different exterior aesthetics can be achieved by adding tints or another coating to the exterior glass pane.
The ability to modulate the sun’s light and heat provides the designer with a controllable heat and light valve for their building; the amount of light and heat coming into the space is tuned depending on the exterior environmental conditions and the needs of the occupants.
By dynamically controlling the light and heat flow, significantly more energy savings can be captured than when using a static façade solution as well as providing enhanced occupant comfort whilst maintaining exterior views. As a result the use of EC glass provides an architect with the ability to design with more glass, thus providing the needed access to daylight and views, without energy or comfort penalty.
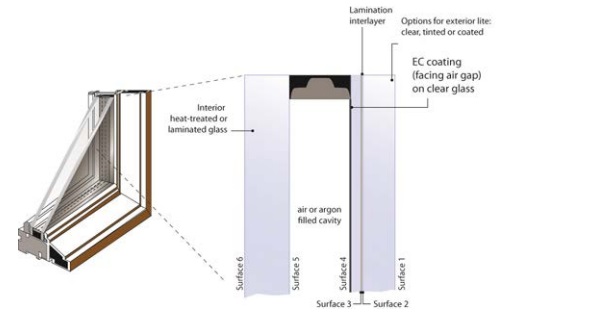
4. DESIGN FLEXIBILITY WITHOUT ENERGY PENALTY
4.1. MORE GLASS WITHOUT ENERGY PENALTY
Because of the ability to dynamically control the heat flows into a building, EC glass can provide additional architectural freedom because more glass can be used, especially on challenging façades, without energy penalty.
For example, modeling work completed recently by Professor Rick Mistrick (Mistrick 2014) at Pennsylvania State University demonstrates that the standard prototypical medium size office building used by PNNL to measure the performance of ASHRAE Standard 90.1 uses less energy in Phoenix when glazed with a 50% window to wall ratio (WWR) of EC than with a 30% WWR of code compliant static glazing (see Figure 4).
When manual blind use is considered, which reduces the energy saved from daylight harvesting, the 50% WWR EC building uses less energy than one at 25% WWR with code compliant glazing.
Note that this prototypical building does not have a good daylighting design and so it does not show the expected optimum profile where the building energy would go down as WWR increases initially because the conductive losses and heat gains are offset by electrical lighting energy savings from daylight harvesting. It is also core load dominated, so the impact of the perimeter is relatively small compared to the core.
4.2. MINIMIZING THE IMPACT OF ORIENTATION
Also, using EC glazing can help minimize the impact of orientation. The optimum orientation for buildings is to put the long axes facing north and south, so as to minimize glazing on the east and west elevations where the sun angle is low and more difficult to shade.
However, sometimes the site and other factors do not allow for the “perfect” orientation, and EC glass can help minimize this impact. Figure 5 shows the results of energy modeling by Prof. Mistrick (Mistrick 2014) on the same PNNL prototypical medium sized office building discussed above as a function of orientation in Phoenix. The energy increase associated with turning the building 45 degrees away from the optimum is much smaller for the EC than with the static glazing systems.
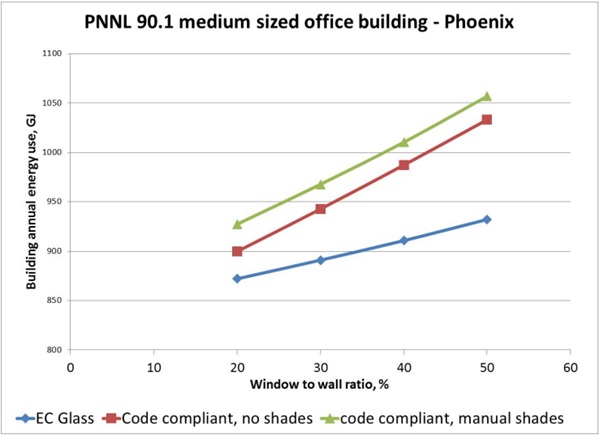
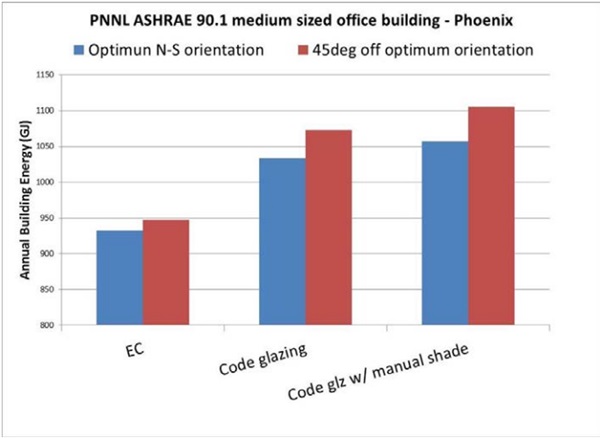
5. LIGHT COLOR QUALITY
Thermal comfort and glare control are not the only aspects of the human comfort equation. The color of the light in a room is important to color rendering of objects and on occupant acceptance as has been seen over the years with compact florescent (CFL) light bulbs, the best of which have a color rendering index (CRI) of around 80-85, but many have had color rendering indices much lower and has led to market acceptance problems.
The color rendering index is a measure of how close the color of light is to the reference “sunlight” spectrum which is given a value of 100. Figure 6 shows the spectral power distributions and respective CRIs of a number of different light sources.
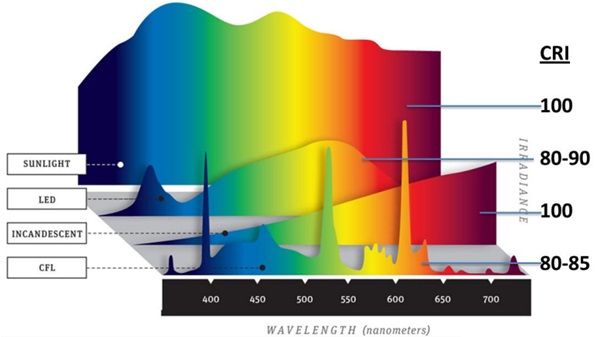
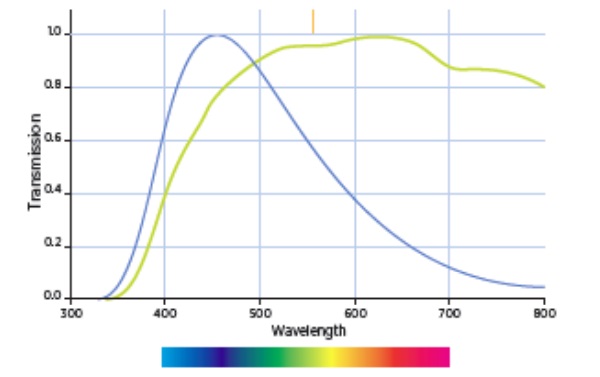
Since EC glass generally tints to a blue-gray color, and previous studies have found that the color rendition of fixed tinted blue glass has caused problems for occupants (Arsenault et al. 2012), the color quality of spaces glazed with EC glass is important to understand.
Studies by John Mardaljevic (University of Loughborough, UK), (Mardaljevic et al 2014; Mardaljevic 2014) has confirmed the empirical observation that the light in spaces glazed with EC glass is essentially the same as in spaces glazed with clear glass as long as a small proportion of the glass is kept in the highest transmittance state.
Figure 7 illustrates the spectral power density of the light coming through EC glass in its fully clear and fully tinted states respectively. Note that the clear state power density is very flat across the visible range which suggests relatively neutral like the spectrum of sunlight in figure 6.
Note that the spectrum of sunlight and daylight changes through the day – starting off more blue in the morning and ending up more red at the end of the day, so even the spectrum of daylight is not static or neutral per se. The color of light coming through the EC in the tinted state is clearly blue, as shown by the peak at around 450nm.
Figure 8 shows schematically how light is combined in the space when transmitted through a façade where the majority of the glass (8 out of 9 panes) is in the fully tinted state and a small amount (1 of 9 panes) is in the clear state.
As can be seen, with the clear state at 60% visible light transmission and the tinted state at 1%T, the bulk of the light transmitted is coming through the single pane in the clear state. As a result the light in the room is dominated by the color of the clear state (about 90% of the light) which is essentially neutral. The resultant spectral power densities of light coming through this, and other combinations of EC panes, are shown in Figure 9.
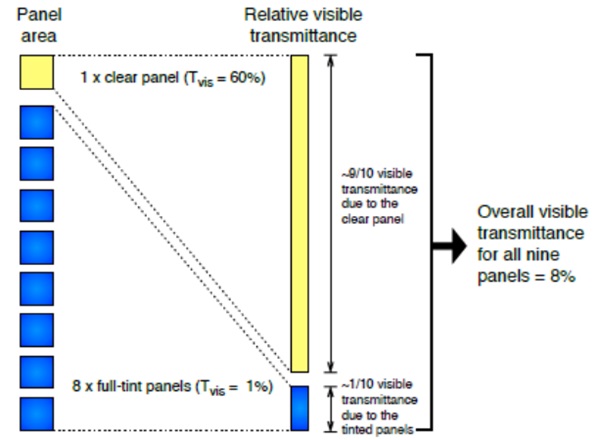
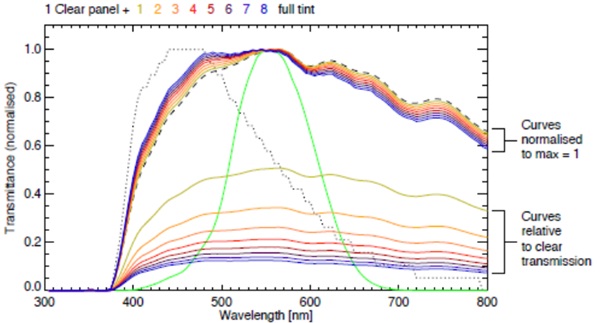
Figure 10 illustrates actual measured spectral power density (SPD) of the light in an office glazed with EC glass at six points in the room – facing the windows, facing the wall opposite the windows, in the four positions where the occupants sit in the office, and compares it to the calculated values based on assuming a 5500K daylight source.
As you can see, the spectra demonstrate that the color of the light is essentially neutral at all positions in the room, even when facing the window and match the theory well. Also the average of the measured color rendering index of the light in the space was 93 which is generally accepted good color rendering.
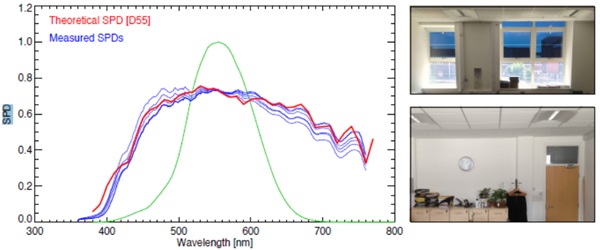
6. ELECTROCHROMIC GLASS DESIGN AND PERFORMANCE ENHANCEMENTS
Electrochromic (EC) glazing which has been commercially available for over ten years and since its initial introduction has undergone significant developments most of which have occurred in the last couple of years. These developments have provided increased additional flexibility for designers and reduced the trade-off architects have had to make between building performance and the design aesthetic.
The most important of these improvements include increased size availability (up to 5’x10’), increased manufacturing volumes, availability of a range of colors and improved reflection characteristics that architects can choose from to match their project color aesthetic (see Figure 11) and the ability to control up to three different areas of a single EC pane as different segments (see Figure 12).
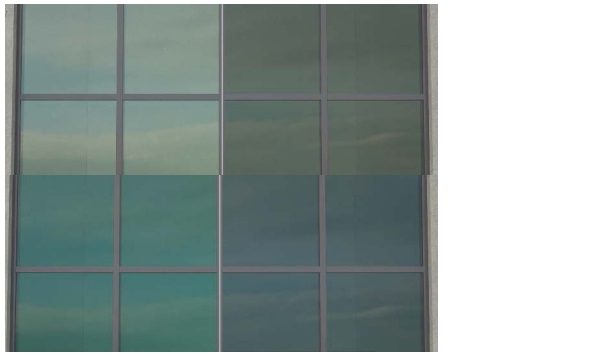
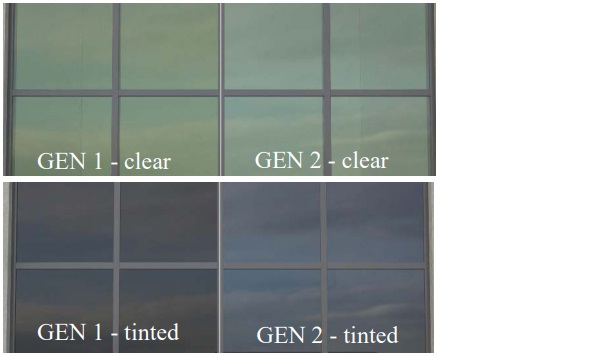
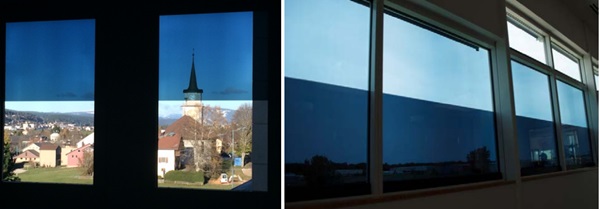
The in-pane zoning feature is essential in floor to ceiling glass to provide for effective co-optimization of glare control, light color quality, daylight admission and energy performance.
For effective glare control, the EC glass needs to be tintable to 1% visible light transmittance, yet if the whole façade is at 1%T, there will be insufficient daylight admission, the lights will have to be turned on and the light color quality will suffer.
With the ability to zone the EC within a pane, optimized performance across multiple parameters can be achieved as well as the architect’s goal of reducing the number of mullions in the framing system.
The following case studies demonstrate how dynamic glass can be used to provide architects with a tool that can expand design possibilities for the use of glass and allow for optimum daylighting and views while compromising neither energy efficiency nor occupant comfort.
7. CASE STUDIES
7.1. “GREEN” GLASS CUBE
The use of glass cube structures in architectural design is all the rage currently. With such a large area of glass, ensuring both energy efficiency and occupant thermal and visual comfort is a challenge, especially in harsh climates.
An example of how EC glazings can meet this challenge is demonstrated in Figure 13 which show EC glass implemented in the Morgan Library expansion at Colorado State University (CSU), a LEED® Silver certified modern glass cube designed by architects at Studiotrope.
Preserving the transparency of the glass building was a key design objective for the project yet the university also wanted to showcase sustainability and efficiency while reflecting the virtues of an open and safe campus.
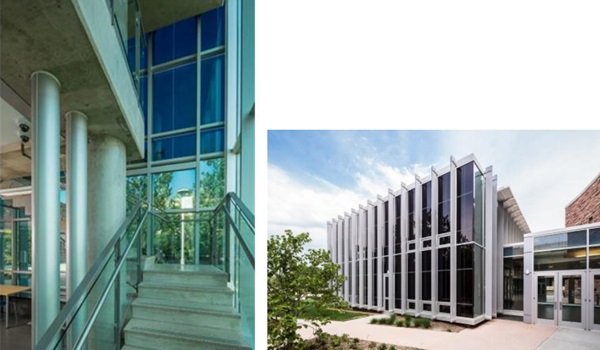
In Colorado the sunlight is intense due to the high altitudes, especially on the west elevation in the afternoon, which is challenging to mitigate using conventional shading methods. In fact, daylight modeling of the space showed that an extremely dense vertical shade solution would be needed to control the western sun, which would have negated the openness and transparency objectives of the design.
As a result, making such a highly glazed design work with the conventional solutions would have been extremely difficult.
A second example of a glass cube design using EC glass is shown in Figure 14. Here Florida East Coast Realty (FECR), the developer of the property, was inspired by Apple’s now iconic cube design of their Manhattan store when planning the addition to their high profile building on Miami’s Brickell Avenue.
However, they were also keenly aware of the negative aspects of highly glazed walls on two sides of the cube in the even more challenging climate of Miami, Florida. EC glass was the solution selected for the project because it could provide the aesthetics of an all glass wall while not sacrificing the visual and thermal comfort for TD Bank, FECR’s sustainable minded tenant.
Even with the two story glazing facing south and west, EC is capable of controlling the light and heat entering the space, blocking 91% of the solar heat gain and 98% of the visible light entering the space keeping the space comfortable for the bank employees and customers even on Miami’s brightest and hottest days.
The EC glass tints and clears automatically based on the amount of solar energy that is present on the exterior -- and desirable on the interior. The automated system allows an entire elevation to be tinted individually or in tandem and they can also be controlled manually with just 2 wall switches.
Note how the color rendering in the space looks neutral because of the combination of panes in the clear state and panes in the tinted state.
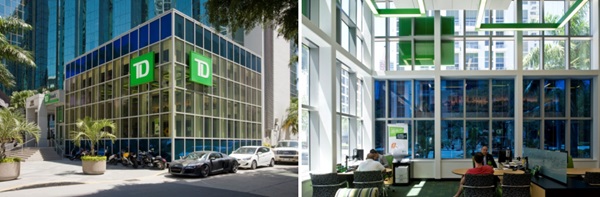
7.2. GREEN HEALTH CARE
Improved daylight admission and views to nature have been shown to increase recovery rates of patients in hospitals and reduce the use of pain medication (see, for example, the foundational study by Ulrich in 1984 (Ulrich 1984) and a summary of the literature developed by Velikov and Janiski commissioned by Guardian Industries (Velikov and Janiski 2012)).
Also, when infection control is so important, the elimination of blinds which can harbor dirt, dust and bacteria is another key benefit for EC in healthcare applications - allowing comfortable daylighting and views with improved infection control. Figure 15 shows some images of a healthcare application at Butler County Medical Center in Nebraska where EC glass has been used to provide an open air feel and an expansive view for the occupants of the new wellness center of the park and golf course it overlooks.
The wellness center features a unique, curved south-facing glass curtain wall that offers beautiful views of a park and golf course. Due to the complex curvature of the curtainwall and non-rectangular shapes, mechanical shades would have been problematic not just because of infection control.
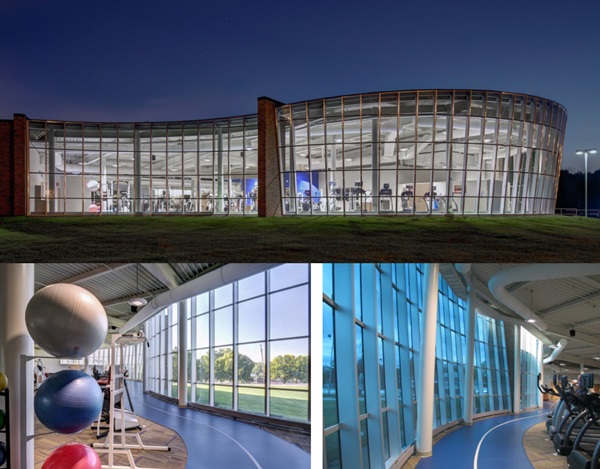
7.3. ENERGY EFFICIENT HISTORIC PRESERVATION
Figure 16 illustrates another application for EC glass where energy saving performance is a requirement along with daylighting, but yet without risk of fading, as well as preservation of the historic building appearance.
Pictured in the figure is one of the renovated skylights containing EC glass over the St. Johnsbury Athenaeum in St. Johnsbury, Vermont. The St. Johnsbury Athenaeum is the oldest art gallery in the U.S. that still maintains its original design.
One distinctive element of the building is its Victorian skylights which flood the gallery’s interiors with natural light and uniquely enhance the viewing experience of the well-known masterpieces such as Albert Bierstadt’s Domes of Yosemite.
Unfortunately, though, natural light poses a threat to the Athenaeum’s art collection and when the skylights deteriorated beyond repair the leadership needed to find a better solution for protecting the gallery’s contents.
Replacing the skylights with traditional static glass would have required the addition of mechanical shades or sun controls that would have not been in keeping with its original appearance as well as severely compromised the appeal of the gallery and the experience of the visitors.
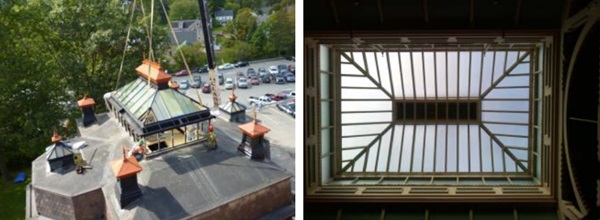
The project architect, John Mesick, specified triple pane EC glass to provide fading protection as well as dynamic light and solar control combined with excellent thermal performance. The U.S. DOE has identified low U-factor windows with dynamic solar control as a key element of zero energy facades (Arasteh et al. 2006) and EC combined in a triple pane provides the optimum energy performance.
Modeling studies have shown that in this configuration EC glass can result in building energy savings greater than 50% compared to a building glazed with single pane glazings and 15% more than a building glazed with standard triple pane low-e (Pease et al. 2010).
The triple pane glazing not only provides excellent thermal insulation performance during Vermont’s cold winters, but it also addresses concerns about humidity levels in the gallery and condensation on the glass.
EC glass also provides excellent protection against fading and sun damage which is important for sustainable design as it can help preserve the lifetime of interior finishing products.
Damage to fabrics and artwork occurs not just from exposure to UV light, but also from exposure to visible light and direct heat. In addition to laminated glass on the inboard lite (needed by code for sloped glazing) which blocks out most of the UV, the EC coatings block the other wavelengths of light that cause fading and reduces direct heating of the objects in the space.
In the fully tinted state, EC glass in this configuration blocks 99% of the light (UV and visible) that causes fading and 87% in its fully clear state1. This compares to ~80% for a double silver low-e glazing with a laminated inboard lite.
In addition, the inboard laminated lite contained textured glass to match the look of the original glass. Mesick stated, “It was critical that the skylight preserve the authentic atmosphere people experience when they visit the Athenaeum. (EC) glass allows us to do that.”
Often in museum and art gallery applications color rendering is an important requirement. Even though in its tinted states EC glazing has a blue hue, when the EC is zoned and controlled appropriately and there is sufficient mixing of light in the space, good color rendering in a space can be achieved to meet the stringent requirements for viewing artwork (Mardaljevic et al. 2014, Mardaljevic 2014).
1 ) Based on the Krochmann damage function which is the weighted average of the wavelengths from 300-500nm that cause fading and damage to materials. Calculations done using the LBNL developed Window 6 program.
7.4. EC GLASS IN RETROFITS: FIXING DESIGN PROBLEMS
EC glass can also be used in retrofit applications to solve heat and light control problems associated with existing designs. Take for example the renowned Kimmel Center for the Performing Arts located in Philadelphia which was designed in 2001 by Raphael Viñoly.
Located on the Avenue of the Arts, it is an architectural icon with a huge barrel vaulted fully glazed roof housing multiple concert halls, theatres and large public spaces. However, the Dorrance H. Hamilton roof top garden, which was originally designed as a rentable space for private functions with amazing panoramic views of the arts center and the surrounding city, was almost uninhabitable from May to September with temperatures up to 50oC (122oF). The Center’s management had to turn away a thousand inquiries annually for renting the space.
In a renovation designed by BLT architects completed last summer, the rooftop garden has been enclosed in a glass box structure. The design employs EC glass in the roof in order to maintain the views of the Center’s vaulted dome and of the city and public spaces, yet maintain a comfortable temperature year round (see Figure 17).
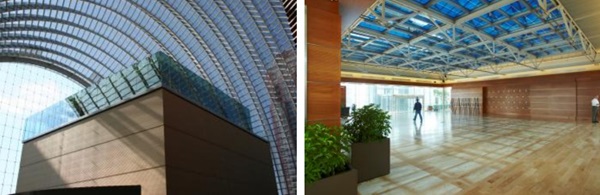
8. CONCLUSIONS
The impact of being able to design with more glass without energy or comfort penalty should not be underestimated. Glass is a key architectural design tool and provides significant design flexibility for architects.
Sustainable design standards and codes recognize the need to use glass to provide occupants with access to daylight and views, because of the positive impact that it has on people’s health and well-being, yet doing so often requires compromising energy performance and at times occupant thermal and visual comfort.
Dynamic glazing can relieve the constraints enforced on designers by static building envelopes when balancing architectural design with occupant comfort and energy efficiency. The improvements in EC glass aesthetics and functionality described herein and the case studies clearly demonstrate that EC glass can provide an elegant façade solution which achieves both the competing goals of high energy performance and access to daylight and views, without compromising occupant thermal and visual comfort or the aesthetics of the façade design.
The ability to maintain architectural design freedom while still providing an energy efficient AND comfortable building is invaluable. This value will continue to increase as energy efficiency targets rise and as the importance of creating people friendly work spaces is further appreciated.
9. ACKNOWLEDGEMENTS
The authors would like to acknowledge Professors John Mardaljevic (Loughborough University) and Rick Mistrick (Pennsylvania State University) for the collaborative work on light color quality and energy modeling of EC glass respectively that they have done with us and the use of data generated in these studies in this paper.
We would also like to acknowledge the following for use of their photographic images: Totaro Photography (Kimmel Center), Daubman Photography (Butler County Healthcare), Kevin Eilbeck Photography (Colorado Springs), Drury Photography (TD Bank), Bob Jenk Photography (St. Johnsbury Athenaeum).
This paper was developed for the fourth BEST Conference Building Enclosure Science & Technology (BEST4), held in Kansas City on April 13-15, 2015.
BEST4 Proceedings